mirror of
https://github.com/RGBCube/serenity
synced 2025-05-17 09:35:07 +00:00
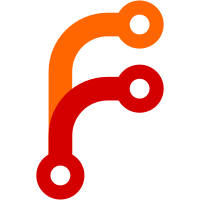
Some occasional cases could cause the accumulator to overflow and have an incorrect result. It would be nice to use a smaller accumulator, but it seems not to be correct. :^( We now cast to i16 to allow 128-bit vectorization to make use of one whole register instead of having to split the loop into multiple. This results in about a 5% reduction in performance in my testing.
1996 lines
110 KiB
C++
1996 lines
110 KiB
C++
/*
|
||
* Copyright (c) 2021, Hunter Salyer <thefalsehonesty@gmail.com>
|
||
* Copyright (c) 2022, Gregory Bertilson <zaggy1024@gmail.com>
|
||
*
|
||
* SPDX-License-Identifier: BSD-2-Clause
|
||
*/
|
||
|
||
#include <AK/IntegralMath.h>
|
||
#include <AK/TypedTransfer.h>
|
||
#include <LibGfx/Size.h>
|
||
#include <LibVideo/Color/CodingIndependentCodePoints.h>
|
||
|
||
#include "Context.h"
|
||
#include "Decoder.h"
|
||
#include "Utilities.h"
|
||
|
||
#if defined(AK_COMPILER_GCC)
|
||
# pragma GCC optimize("O3")
|
||
#endif
|
||
|
||
namespace Video::VP9 {
|
||
|
||
Decoder::Decoder()
|
||
: m_parser(make<Parser>(*this))
|
||
{
|
||
}
|
||
|
||
DecoderErrorOr<void> Decoder::receive_sample(ReadonlyBytes chunk_data)
|
||
{
|
||
auto superframe_sizes = m_parser->parse_superframe_sizes(chunk_data);
|
||
|
||
if (superframe_sizes.is_empty()) {
|
||
return decode_frame(chunk_data);
|
||
}
|
||
|
||
size_t offset = 0;
|
||
|
||
for (auto superframe_size : superframe_sizes) {
|
||
auto checked_size = Checked<size_t>(superframe_size);
|
||
checked_size += offset;
|
||
if (checked_size.has_overflow() || checked_size.value() > chunk_data.size())
|
||
return DecoderError::with_description(DecoderErrorCategory::Corrupted, "Superframe size invalid"sv);
|
||
auto frame_data = chunk_data.slice(offset, superframe_size);
|
||
TRY(decode_frame(frame_data));
|
||
offset = checked_size.value();
|
||
}
|
||
|
||
return {};
|
||
}
|
||
|
||
DecoderErrorOr<void> Decoder::decode_frame(ReadonlyBytes frame_data)
|
||
{
|
||
// 1. The syntax elements for the coded frame are extracted as specified in sections 6 and 7. The syntax
|
||
// tables include function calls indicating when the block decode processes should be triggered.
|
||
auto frame_context = TRY(m_parser->parse_frame(frame_data));
|
||
|
||
// 2. If loop_filter_level is not equal to 0, the loop filter process as specified in section 8.8 is invoked once the
|
||
// coded frame has been decoded.
|
||
// FIXME: Implement loop filtering.
|
||
|
||
// 3. If all of the following conditions are true, PrevSegmentIds[ row ][ col ] is set equal to
|
||
// SegmentIds[ row ][ col ] for row = 0..MiRows-1, for col = 0..MiCols-1:
|
||
// − show_existing_frame is equal to 0,
|
||
// − segmentation_enabled is equal to 1,
|
||
// − segmentation_update_map is equal to 1.
|
||
// This is handled by update_reference_frames.
|
||
|
||
// 4. The output process as specified in section 8.9 is invoked.
|
||
if (frame_context.shows_a_frame())
|
||
TRY(create_video_frame(frame_context));
|
||
|
||
// 5. The reference frame update process as specified in section 8.10 is invoked.
|
||
TRY(update_reference_frames(frame_context));
|
||
return {};
|
||
}
|
||
|
||
inline CodingIndependentCodePoints get_cicp_color_space(FrameContext const& frame_context)
|
||
{
|
||
ColorPrimaries color_primaries;
|
||
TransferCharacteristics transfer_characteristics;
|
||
MatrixCoefficients matrix_coefficients;
|
||
|
||
switch (frame_context.color_config.color_space) {
|
||
case ColorSpace::Unknown:
|
||
color_primaries = ColorPrimaries::Unspecified;
|
||
transfer_characteristics = TransferCharacteristics::Unspecified;
|
||
matrix_coefficients = MatrixCoefficients::Unspecified;
|
||
break;
|
||
case ColorSpace::Bt601:
|
||
color_primaries = ColorPrimaries::BT601;
|
||
transfer_characteristics = TransferCharacteristics::BT601;
|
||
matrix_coefficients = MatrixCoefficients::BT601;
|
||
break;
|
||
case ColorSpace::Bt709:
|
||
color_primaries = ColorPrimaries::BT709;
|
||
transfer_characteristics = TransferCharacteristics::BT709;
|
||
matrix_coefficients = MatrixCoefficients::BT709;
|
||
break;
|
||
case ColorSpace::Smpte170:
|
||
// https://www.kernel.org/doc/html/v4.9/media/uapi/v4l/pixfmt-007.html#colorspace-smpte-170m-v4l2-colorspace-smpte170m
|
||
color_primaries = ColorPrimaries::BT601;
|
||
transfer_characteristics = TransferCharacteristics::BT709;
|
||
matrix_coefficients = MatrixCoefficients::BT601;
|
||
break;
|
||
case ColorSpace::Smpte240:
|
||
color_primaries = ColorPrimaries::SMPTE240;
|
||
transfer_characteristics = TransferCharacteristics::SMPTE240;
|
||
matrix_coefficients = MatrixCoefficients::SMPTE240;
|
||
break;
|
||
case ColorSpace::Bt2020:
|
||
color_primaries = ColorPrimaries::BT2020;
|
||
// Bit depth doesn't actually matter to our transfer functions since we
|
||
// convert in floats of range 0-1 (for now?), but just for correctness set
|
||
// the TC to match the bit depth here.
|
||
if (frame_context.color_config.bit_depth == 12)
|
||
transfer_characteristics = TransferCharacteristics::BT2020BitDepth12;
|
||
else if (frame_context.color_config.bit_depth == 10)
|
||
transfer_characteristics = TransferCharacteristics::BT2020BitDepth10;
|
||
else
|
||
transfer_characteristics = TransferCharacteristics::BT709;
|
||
matrix_coefficients = MatrixCoefficients::BT2020NonConstantLuminance;
|
||
break;
|
||
case ColorSpace::RGB:
|
||
color_primaries = ColorPrimaries::BT709;
|
||
transfer_characteristics = TransferCharacteristics::Linear;
|
||
matrix_coefficients = MatrixCoefficients::Identity;
|
||
break;
|
||
case ColorSpace::Reserved:
|
||
VERIFY_NOT_REACHED();
|
||
break;
|
||
}
|
||
|
||
return { color_primaries, transfer_characteristics, matrix_coefficients, frame_context.color_config.color_range };
|
||
}
|
||
|
||
DecoderErrorOr<void> Decoder::create_video_frame(FrameContext const& frame_context)
|
||
{
|
||
// (8.9) Output process
|
||
|
||
// FIXME: If show_existing_frame is set, output from FrameStore[frame_to_show_map_index] here instead.
|
||
if (frame_context.shows_existing_frame()) {
|
||
dbgln("FIXME: Show an existing reference frame.");
|
||
}
|
||
|
||
// FIXME: The math isn't entirely accurate to spec. output_uv_size is probably incorrect for certain
|
||
// sizes, as the spec seems to prefer that the halved sizes be ceiled.
|
||
u32 decoded_y_width = frame_context.decoded_size(false).width();
|
||
auto decoded_uv_width = frame_context.decoded_size(true).width();
|
||
Gfx::Size<u32> output_y_size = frame_context.size();
|
||
auto subsampling_x = frame_context.color_config.subsampling_x;
|
||
auto subsampling_y = frame_context.color_config.subsampling_y;
|
||
Gfx::Size<u32> output_uv_size = {
|
||
y_size_to_uv_size(subsampling_x, output_y_size.width()),
|
||
y_size_to_uv_size(subsampling_y, output_y_size.height()),
|
||
};
|
||
Array<FixedArray<u16>, 3> output_buffers = {
|
||
DECODER_TRY_ALLOC(FixedArray<u16>::create(output_y_size.width() * output_y_size.height())),
|
||
DECODER_TRY_ALLOC(FixedArray<u16>::create(output_uv_size.width() * output_uv_size.height())),
|
||
DECODER_TRY_ALLOC(FixedArray<u16>::create(output_uv_size.width() * output_uv_size.height())),
|
||
};
|
||
for (u8 plane = 0; plane < 3; plane++) {
|
||
auto& buffer = output_buffers[plane];
|
||
auto decoded_width = plane == 0 ? decoded_y_width : decoded_uv_width;
|
||
auto output_size = plane == 0 ? output_y_size : output_uv_size;
|
||
auto const& decoded_buffer = get_output_buffer(plane);
|
||
|
||
for (u32 row = 0; row < output_size.height(); row++) {
|
||
memcpy(
|
||
buffer.data() + row * output_size.width(),
|
||
decoded_buffer.data() + row * decoded_width,
|
||
output_size.width() * sizeof(*buffer.data()));
|
||
}
|
||
}
|
||
|
||
auto frame = DECODER_TRY_ALLOC(adopt_nonnull_own_or_enomem(new (nothrow) SubsampledYUVFrame(
|
||
{ output_y_size.width(), output_y_size.height() },
|
||
frame_context.color_config.bit_depth, get_cicp_color_space(frame_context),
|
||
subsampling_x, subsampling_y,
|
||
output_buffers[0], output_buffers[1], output_buffers[2])));
|
||
m_video_frame_queue.enqueue(move(frame));
|
||
|
||
return {};
|
||
}
|
||
|
||
DecoderErrorOr<void> Decoder::allocate_buffers(FrameContext const& frame_context)
|
||
{
|
||
for (size_t plane = 0; plane < 3; plane++) {
|
||
auto size = frame_context.decoded_size(plane > 0);
|
||
|
||
auto& output_buffer = get_output_buffer(plane);
|
||
output_buffer.clear_with_capacity();
|
||
DECODER_TRY_ALLOC(output_buffer.try_resize_and_keep_capacity(size.width() * size.height()));
|
||
}
|
||
return {};
|
||
}
|
||
|
||
Vector<u16>& Decoder::get_output_buffer(u8 plane)
|
||
{
|
||
return m_output_buffers[plane];
|
||
}
|
||
|
||
DecoderErrorOr<NonnullOwnPtr<VideoFrame>> Decoder::get_decoded_frame()
|
||
{
|
||
if (m_video_frame_queue.is_empty())
|
||
return DecoderError::format(DecoderErrorCategory::NeedsMoreInput, "No video frame in queue.");
|
||
|
||
return m_video_frame_queue.dequeue();
|
||
}
|
||
|
||
template<typename T>
|
||
static inline i32 rounded_right_shift(T value, u8 bits)
|
||
{
|
||
value = (value + static_cast<T>(1u << (bits - 1u))) >> bits;
|
||
return static_cast<i32>(value);
|
||
}
|
||
|
||
u8 Decoder::merge_prob(u8 pre_prob, u32 count_0, u32 count_1, u8 count_sat, u8 max_update_factor)
|
||
{
|
||
auto total_decode_count = count_0 + count_1;
|
||
u8 prob = 128;
|
||
if (total_decode_count != 0) {
|
||
prob = static_cast<u8>(clip_3(1u, 255u, (count_0 * 256 + (total_decode_count >> 1)) / total_decode_count));
|
||
}
|
||
auto count = min(total_decode_count, count_sat);
|
||
auto factor = (max_update_factor * count) / count_sat;
|
||
return rounded_right_shift(pre_prob * (256 - factor) + (prob * factor), 8);
|
||
}
|
||
|
||
u32 Decoder::merge_probs(int const* tree, int index, u8* probs, u32* counts, u8 count_sat, u8 max_update_factor)
|
||
{
|
||
auto s = tree[index];
|
||
auto left_count = (s <= 0) ? counts[-s] : merge_probs(tree, s, probs, counts, count_sat, max_update_factor);
|
||
auto r = tree[index + 1];
|
||
auto right_count = (r <= 0) ? counts[-r] : merge_probs(tree, r, probs, counts, count_sat, max_update_factor);
|
||
probs[index >> 1] = merge_prob(probs[index >> 1], left_count, right_count, count_sat, max_update_factor);
|
||
return left_count + right_count;
|
||
}
|
||
|
||
DecoderErrorOr<void> Decoder::adapt_coef_probs(FrameContext const& frame_context)
|
||
{
|
||
u8 update_factor;
|
||
if (!frame_context.is_inter_predicted() || m_parser->m_previous_frame_type != FrameType::KeyFrame)
|
||
update_factor = 112;
|
||
else
|
||
update_factor = 128;
|
||
|
||
for (size_t t = 0; t < 4; t++) {
|
||
for (size_t i = 0; i < 2; i++) {
|
||
for (size_t j = 0; j < 2; j++) {
|
||
for (size_t k = 0; k < 6; k++) {
|
||
size_t max_l = (k == 0) ? 3 : 6;
|
||
for (size_t l = 0; l < max_l; l++) {
|
||
auto& coef_probs = m_parser->m_probability_tables->coef_probs()[t][i][j][k][l];
|
||
merge_probs(small_token_tree, 2, coef_probs,
|
||
frame_context.counter->m_counts_token[t][i][j][k][l],
|
||
24, update_factor);
|
||
merge_probs(binary_tree, 0, coef_probs,
|
||
frame_context.counter->m_counts_more_coefs[t][i][j][k][l],
|
||
24, update_factor);
|
||
}
|
||
}
|
||
}
|
||
}
|
||
}
|
||
|
||
return {};
|
||
}
|
||
|
||
#define ADAPT_PROB_TABLE(name, size) \
|
||
do { \
|
||
for (size_t i = 0; i < (size); i++) { \
|
||
auto table = probs.name##_prob(); \
|
||
table[i] = adapt_prob(table[i], counter.m_counts_##name[i]); \
|
||
} \
|
||
} while (0)
|
||
|
||
#define ADAPT_TREE(tree_name, prob_name, count_name, size) \
|
||
do { \
|
||
for (size_t i = 0; i < (size); i++) { \
|
||
adapt_probs(tree_name##_tree, probs.prob_name##_probs()[i], counter.m_counts_##count_name[i]); \
|
||
} \
|
||
} while (0)
|
||
|
||
DecoderErrorOr<void> Decoder::adapt_non_coef_probs(FrameContext const& frame_context)
|
||
{
|
||
auto& probs = *m_parser->m_probability_tables;
|
||
auto& counter = *frame_context.counter;
|
||
ADAPT_PROB_TABLE(is_inter, IS_INTER_CONTEXTS);
|
||
ADAPT_PROB_TABLE(comp_mode, COMP_MODE_CONTEXTS);
|
||
ADAPT_PROB_TABLE(comp_ref, REF_CONTEXTS);
|
||
for (size_t i = 0; i < REF_CONTEXTS; i++) {
|
||
for (size_t j = 0; j < 2; j++)
|
||
probs.single_ref_prob()[i][j] = adapt_prob(probs.single_ref_prob()[i][j], counter.m_counts_single_ref[i][j]);
|
||
}
|
||
ADAPT_TREE(inter_mode, inter_mode, inter_mode, INTER_MODE_CONTEXTS);
|
||
ADAPT_TREE(intra_mode, y_mode, intra_mode, BLOCK_SIZE_GROUPS);
|
||
ADAPT_TREE(intra_mode, uv_mode, uv_mode, INTRA_MODES);
|
||
ADAPT_TREE(partition, partition, partition, PARTITION_CONTEXTS);
|
||
ADAPT_PROB_TABLE(skip, SKIP_CONTEXTS);
|
||
if (frame_context.interpolation_filter == Switchable) {
|
||
ADAPT_TREE(interp_filter, interp_filter, interp_filter, INTERP_FILTER_CONTEXTS);
|
||
}
|
||
if (frame_context.transform_mode == TransformMode::Select) {
|
||
for (size_t i = 0; i < TX_SIZE_CONTEXTS; i++) {
|
||
auto& tx_probs = probs.tx_probs();
|
||
auto& tx_counts = counter.m_counts_tx_size;
|
||
adapt_probs(tx_size_8_tree, tx_probs[Transform_8x8][i], tx_counts[Transform_8x8][i]);
|
||
adapt_probs(tx_size_16_tree, tx_probs[Transform_16x16][i], tx_counts[Transform_16x16][i]);
|
||
adapt_probs(tx_size_32_tree, tx_probs[Transform_32x32][i], tx_counts[Transform_32x32][i]);
|
||
}
|
||
}
|
||
adapt_probs(mv_joint_tree, probs.mv_joint_probs(), counter.m_counts_mv_joint);
|
||
for (size_t i = 0; i < 2; i++) {
|
||
probs.mv_sign_prob()[i] = adapt_prob(probs.mv_sign_prob()[i], counter.m_counts_mv_sign[i]);
|
||
adapt_probs(mv_class_tree, probs.mv_class_probs()[i], counter.m_counts_mv_class[i]);
|
||
probs.mv_class0_bit_prob()[i] = adapt_prob(probs.mv_class0_bit_prob()[i], counter.m_counts_mv_class0_bit[i]);
|
||
for (size_t j = 0; j < MV_OFFSET_BITS; j++)
|
||
probs.mv_bits_prob()[i][j] = adapt_prob(probs.mv_bits_prob()[i][j], counter.m_counts_mv_bits[i][j]);
|
||
for (size_t j = 0; j < CLASS0_SIZE; j++)
|
||
adapt_probs(mv_fr_tree, probs.mv_class0_fr_probs()[i][j], counter.m_counts_mv_class0_fr[i][j]);
|
||
adapt_probs(mv_fr_tree, probs.mv_fr_probs()[i], counter.m_counts_mv_fr[i]);
|
||
if (frame_context.high_precision_motion_vectors_allowed) {
|
||
probs.mv_class0_hp_prob()[i] = adapt_prob(probs.mv_class0_hp_prob()[i], counter.m_counts_mv_class0_hp[i]);
|
||
probs.mv_hp_prob()[i] = adapt_prob(probs.mv_hp_prob()[i], counter.m_counts_mv_hp[i]);
|
||
}
|
||
}
|
||
return {};
|
||
}
|
||
|
||
void Decoder::adapt_probs(int const* tree, u8* probs, u32* counts)
|
||
{
|
||
merge_probs(tree, 0, probs, counts, COUNT_SAT, MAX_UPDATE_FACTOR);
|
||
}
|
||
|
||
u8 Decoder::adapt_prob(u8 prob, u32 counts[2])
|
||
{
|
||
return merge_prob(prob, counts[0], counts[1], COUNT_SAT, MAX_UPDATE_FACTOR);
|
||
}
|
||
|
||
DecoderErrorOr<void> Decoder::predict_intra(u8 plane, BlockContext const& block_context, u32 x, u32 y, bool have_left, bool have_above, bool not_on_right, TransformSize tx_size, u32 block_index)
|
||
{
|
||
auto& frame_buffer = get_output_buffer(plane);
|
||
|
||
// 8.5.1 Intra prediction process
|
||
|
||
// The intra prediction process is invoked for intra coded blocks to predict a part of the block corresponding to a
|
||
// transform block. When the transform size is smaller than the block size, this process can be invoked multiple
|
||
// times within a single block for the same plane, and the invocations are in raster order within the block.
|
||
|
||
// The variable mode is specified by:
|
||
// 1. If plane is greater than 0, mode is set equal to uv_mode.
|
||
// 2. Otherwise, if MiSize is greater than or equal to BLOCK_8X8, mode is set equal to y_mode.
|
||
// 3. Otherwise, mode is set equal to sub_modes[ blockIdx ].
|
||
PredictionMode mode;
|
||
if (plane > 0)
|
||
mode = block_context.uv_prediction_mode;
|
||
else if (block_context.size >= Block_8x8)
|
||
mode = block_context.y_prediction_mode();
|
||
else
|
||
mode = block_context.sub_block_prediction_modes[block_index];
|
||
|
||
// The variable log2Size specifying the base 2 logarithm of the width of the transform block is set equal to txSz + 2.
|
||
u8 log2_of_block_size = tx_size + 2;
|
||
// The variable size is set equal to 1 << log2Size.
|
||
u8 block_size = 1 << log2_of_block_size;
|
||
|
||
// The variable maxX is set equal to (MiCols * 8) - 1.
|
||
// The variable maxY is set equal to (MiRows * 8) - 1.
|
||
// If plane is greater than 0, then:
|
||
// − maxX is set equal to ((MiCols * 8) >> subsampling_x) - 1.
|
||
// − maxY is set equal to ((MiRows * 8) >> subsampling_y) - 1.
|
||
auto output_size = block_context.frame_context.decoded_size(plane > 0);
|
||
auto max_x = output_size.width() - 1;
|
||
auto max_y = output_size.height() - 1;
|
||
|
||
auto const frame_buffer_at = [&](u32 row, u32 column) -> u16& {
|
||
return frame_buffer[row * output_size.width() + column];
|
||
};
|
||
|
||
// The array aboveRow[ i ] for i = 0..size-1 is specified by:
|
||
// ..
|
||
// The array aboveRow[ i ] for i = size..2*size-1 is specified by:
|
||
// ..
|
||
// The array aboveRow[ i ] for i = -1 is specified by:
|
||
// ..
|
||
|
||
// NOTE: above_row is an array ranging from 0 to (2*block_size).
|
||
// There are three sections to the array:
|
||
// - [0]
|
||
// - [1 .. block_size]
|
||
// - [block_size + 1 .. block_size * 2]
|
||
// The array indices must be offset by 1 to accommodate index -1.
|
||
Array<Intermediate, maximum_block_dimensions * 2 + 1> above_row;
|
||
auto above_row_at = [&](i32 index) -> Intermediate& {
|
||
return above_row[index + 1];
|
||
};
|
||
|
||
// NOTE: This value is pre-calculated since it is reused in spec below.
|
||
// Use this to replace spec text "(1<<(BitDepth-1))".
|
||
Intermediate half_sample_value = (1 << (block_context.frame_context.color_config.bit_depth - 1));
|
||
|
||
// The array aboveRow[ i ] for i = 0..size-1 is specified by:
|
||
if (!have_above) {
|
||
// 1. If haveAbove is equal to 0, aboveRow[ i ] is set equal to (1<<(BitDepth-1)) - 1.
|
||
// FIXME: Use memset?
|
||
for (auto i = 0u; i < block_size; i++)
|
||
above_row_at(i) = half_sample_value - 1;
|
||
} else {
|
||
// 2. Otherwise, aboveRow[ i ] is set equal to CurrFrame[ plane ][ y-1 ][ Min(maxX, x+i) ].
|
||
for (auto i = 0u; i < block_size; i++)
|
||
above_row_at(i) = frame_buffer_at(y - 1, min(max_x, x + i));
|
||
}
|
||
|
||
// The array aboveRow[ i ] for i = size..2*size-1 is specified by:
|
||
if (have_above && not_on_right && tx_size == Transform_4x4) {
|
||
// 1. If haveAbove is equal to 1 and notOnRight is equal to 1 and txSz is equal to 0,
|
||
// aboveRow[ i ] is set equal to CurrFrame[ plane ][ y-1 ][ Min(maxX, x+i) ].
|
||
for (auto i = block_size; i < block_size * 2; i++)
|
||
above_row_at(i) = frame_buffer_at(y - 1, min(max_x, x + i));
|
||
} else {
|
||
// 2. Otherwise, aboveRow[ i ] is set equal to aboveRow[ size-1 ].
|
||
for (auto i = block_size; i < block_size * 2; i++)
|
||
above_row_at(i) = above_row_at(block_size - 1);
|
||
}
|
||
|
||
// The array aboveRow[ i ] for i = -1 is specified by:
|
||
if (have_above && have_left) {
|
||
// 1. If haveAbove is equal to 1 and haveLeft is equal to 1, aboveRow[ -1 ] is set equal to
|
||
// CurrFrame[ plane ][ y-1 ][ Min(maxX, x-1) ].
|
||
above_row_at(-1) = frame_buffer_at(y - 1, min(max_x, x - 1));
|
||
} else if (have_above) {
|
||
// 2. Otherwise if haveAbove is equal to 1, aboveRow[ -1] is set equal to (1<<(BitDepth-1)) + 1.
|
||
above_row_at(-1) = half_sample_value + 1;
|
||
} else {
|
||
// 3. Otherwise, aboveRow[ -1 ] is set equal to (1<<(BitDepth-1)) - 1
|
||
above_row_at(-1) = half_sample_value - 1;
|
||
}
|
||
|
||
// The array leftCol[ i ] for i = 0..size-1 is specified by:
|
||
Array<Intermediate, maximum_block_dimensions> left_column;
|
||
if (have_left) {
|
||
// − If haveLeft is equal to 1, leftCol[ i ] is set equal to CurrFrame[ plane ][ Min(maxY, y+i) ][ x-1 ].
|
||
for (auto i = 0u; i < block_size; i++)
|
||
left_column[i] = frame_buffer_at(min(max_y, y + i), x - 1);
|
||
} else {
|
||
// − Otherwise, leftCol[ i ] is set equal to (1<<(BitDepth-1)) + 1.
|
||
for (auto i = 0u; i < block_size; i++)
|
||
left_column[i] = half_sample_value + 1;
|
||
}
|
||
|
||
// A 2D array named pred containing the intra predicted samples is constructed as follows:
|
||
Array<Intermediate, maximum_block_size> predicted_samples;
|
||
auto const predicted_sample_at = [&](u32 row, u32 column) -> Intermediate& {
|
||
return predicted_samples[row * block_size + column];
|
||
};
|
||
|
||
// FIXME: One of the two below should be a simple memcpy of 1D arrays.
|
||
switch (mode) {
|
||
case PredictionMode::VPred:
|
||
// − If mode is equal to V_PRED, pred[ i ][ j ] is set equal to aboveRow[ j ] with j = 0..size-1 and i = 0..size-1
|
||
// (each row of the block is filled with a copy of aboveRow).
|
||
for (auto j = 0u; j < block_size; j++) {
|
||
for (auto i = 0u; i < block_size; i++)
|
||
predicted_sample_at(i, j) = above_row_at(j);
|
||
}
|
||
break;
|
||
case PredictionMode::HPred:
|
||
// − Otherwise if mode is equal to H_PRED, pred[ i ][ j ] is set equal to leftCol[ i ] with j = 0..size-1 and i =
|
||
// 0..size-1 (each column of the block is filled with a copy of leftCol).
|
||
for (auto j = 0u; j < block_size; j++) {
|
||
for (auto i = 0u; i < block_size; i++)
|
||
predicted_sample_at(i, j) = left_column[i];
|
||
}
|
||
break;
|
||
case PredictionMode::D207Pred:
|
||
// − Otherwise if mode is equal to D207_PRED, the following applies:
|
||
// 1. pred[ size - 1 ][ j ] = leftCol[ size - 1] for j = 0..size-1
|
||
for (auto j = 0u; j < block_size; j++)
|
||
predicted_sample_at(block_size - 1, j) = left_column[block_size - 1];
|
||
// 2. pred[ i ][ 0 ] = Round2( leftCol[ i ] + leftCol[ i + 1 ], 1 ) for i = 0..size-2
|
||
for (auto i = 0u; i < block_size - 1u; i++)
|
||
predicted_sample_at(i, 0) = rounded_right_shift(left_column[i] + left_column[i + 1], 1);
|
||
// 3. pred[ i ][ 1 ] = Round2( leftCol[ i ] + 2 * leftCol[ i + 1 ] + leftCol[ i + 2 ], 2 ) for i = 0..size-3
|
||
for (auto i = 0u; i < block_size - 2u; i++)
|
||
predicted_sample_at(i, 1) = rounded_right_shift(left_column[i] + (2 * left_column[i + 1]) + left_column[i + 2], 2);
|
||
// 4. pred[ size - 2 ][ 1 ] = Round2( leftCol[ size - 2 ] + 3 * leftCol[ size - 1 ], 2 )
|
||
predicted_sample_at(block_size - 2, 1) = rounded_right_shift(left_column[block_size - 2] + (3 * left_column[block_size - 1]), 2);
|
||
// 5. pred[ i ][ j ] = pred[ i + 1 ][ j - 2 ] for i = (size-2)..0, for j = 2..size-1
|
||
// NOTE – In the last step i iterates in reverse order.
|
||
for (auto i = block_size - 2u;;) {
|
||
for (auto j = 2u; j < block_size; j++)
|
||
predicted_sample_at(i, j) = predicted_sample_at(i + 1, j - 2);
|
||
if (i == 0)
|
||
break;
|
||
i--;
|
||
}
|
||
break;
|
||
case PredictionMode::D45Pred:
|
||
// Otherwise if mode is equal to D45_PRED,
|
||
// for i = 0..size-1, for j = 0..size-1.
|
||
for (auto i = 0u; i < block_size; i++) {
|
||
for (auto j = 0; j < block_size; j++) {
|
||
// pred[ i ][ j ] is set equal to (i + j + 2 < size * 2) ?
|
||
if (i + j + 2 < block_size * 2)
|
||
// Round2( aboveRow[ i + j ] + aboveRow[ i + j + 1 ] * 2 + aboveRow[ i + j + 2 ], 2 ) :
|
||
predicted_sample_at(i, j) = rounded_right_shift(above_row_at(i + j) + above_row_at(i + j + 1) * 2 + above_row_at(i + j + 2), 2);
|
||
else
|
||
// aboveRow[ 2 * size - 1 ]
|
||
predicted_sample_at(i, j) = above_row_at(2 * block_size - 1);
|
||
}
|
||
}
|
||
break;
|
||
case PredictionMode::D63Pred:
|
||
// Otherwise if mode is equal to D63_PRED,
|
||
for (auto i = 0u; i < block_size; i++) {
|
||
for (auto j = 0u; j < block_size; j++) {
|
||
// i/2 + j
|
||
auto row_index = (i / 2) + j;
|
||
// pred[ i ][ j ] is set equal to (i & 1) ?
|
||
if (i & 1)
|
||
// Round2( aboveRow[ i/2 + j ] + aboveRow[ i/2 + j + 1 ] * 2 + aboveRow[ i/2 + j + 2 ], 2 ) :
|
||
predicted_sample_at(i, j) = rounded_right_shift(above_row_at(row_index) + above_row_at(row_index + 1) * 2 + above_row_at(row_index + 2), 2);
|
||
else
|
||
// Round2( aboveRow[ i/2 + j ] + aboveRow[ i/2 + j + 1 ], 1 ) for i = 0..size-1, for j = 0..size-1.
|
||
predicted_sample_at(i, j) = rounded_right_shift(above_row_at(row_index) + above_row_at(row_index + 1), 1);
|
||
}
|
||
}
|
||
break;
|
||
case PredictionMode::D117Pred:
|
||
// Otherwise if mode is equal to D117_PRED, the following applies:
|
||
// 1. pred[ 0 ][ j ] = Round2( aboveRow[ j - 1 ] + aboveRow[ j ], 1 ) for j = 0..size-1
|
||
for (auto j = 0; j < block_size; j++)
|
||
predicted_sample_at(0, j) = rounded_right_shift(above_row_at(j - 1) + above_row_at(j), 1);
|
||
// 2. pred[ 1 ][ 0 ] = Round2( leftCol[ 0 ] + 2 * aboveRow[ -1 ] + aboveRow[ 0 ], 2 )
|
||
predicted_sample_at(1, 0) = rounded_right_shift(left_column[0] + 2 * above_row_at(-1) + above_row_at(0), 2);
|
||
// 3. pred[ 1 ][ j ] = Round2( aboveRow[ j - 2 ] + 2 * aboveRow[ j - 1 ] + aboveRow[ j ], 2 ) for j = 1..size-1
|
||
for (auto j = 1; j < block_size; j++)
|
||
predicted_sample_at(1, j) = rounded_right_shift(above_row_at(j - 2) + 2 * above_row_at(j - 1) + above_row_at(j), 2);
|
||
// 4. pred[ 2 ][ 0 ] = Round2( aboveRow[ -1 ] + 2 * leftCol[ 0 ] + leftCol[ 1 ], 2 )
|
||
predicted_sample_at(2, 0) = rounded_right_shift(above_row_at(-1) + 2 * left_column[0] + left_column[1], 2);
|
||
// 5. pred[ i ][ 0 ] = Round2( leftCol[ i - 3 ] + 2 * leftCol[ i - 2 ] + leftCol[ i - 1 ], 2 ) for i = 3..size-1
|
||
for (auto i = 3u; i < block_size; i++)
|
||
predicted_sample_at(i, 0) = rounded_right_shift(left_column[i - 3] + 2 * left_column[i - 2] + left_column[i - 1], 2);
|
||
// 6. pred[ i ][ j ] = pred[ i - 2 ][ j - 1 ] for i = 2..size-1, for j = 1..size-1
|
||
for (auto i = 2u; i < block_size; i++) {
|
||
for (auto j = 1u; j < block_size; j++)
|
||
predicted_sample_at(i, j) = predicted_sample_at(i - 2, j - 1);
|
||
}
|
||
break;
|
||
case PredictionMode::D135Pred:
|
||
// Otherwise if mode is equal to D135_PRED, the following applies:
|
||
// 1. pred[ 0 ][ 0 ] = Round2( leftCol[ 0 ] + 2 * aboveRow[ -1 ] + aboveRow[ 0 ], 2 )
|
||
predicted_sample_at(0, 0) = rounded_right_shift(left_column[0] + 2 * above_row_at(-1) + above_row_at(0), 2);
|
||
// 2. pred[ 0 ][ j ] = Round2( aboveRow[ j - 2 ] + 2 * aboveRow[ j - 1 ] + aboveRow[ j ], 2 ) for j = 1..size-1
|
||
for (auto j = 1; j < block_size; j++)
|
||
predicted_sample_at(0, j) = rounded_right_shift(above_row_at(j - 2) + 2 * above_row_at(j - 1) + above_row_at(j), 2);
|
||
// 3. pred[ 1 ][ 0 ] = Round2( aboveRow [ -1 ] + 2 * leftCol[ 0 ] + leftCol[ 1 ], 2 ) for i = 1..size-1
|
||
predicted_sample_at(1, 0) = rounded_right_shift(above_row_at(-1) + 2 * left_column[0] + left_column[1], 2);
|
||
// 4. pred[ i ][ 0 ] = Round2( leftCol[ i - 2 ] + 2 * leftCol[ i - 1 ] + leftCol[ i ], 2 ) for i = 2..size-1
|
||
for (auto i = 2u; i < block_size; i++)
|
||
predicted_sample_at(i, 0) = rounded_right_shift(left_column[i - 2] + 2 * left_column[i - 1] + left_column[i], 2);
|
||
// 5. pred[ i ][ j ] = pred[ i - 1 ][ j - 1 ] for i = 1..size-1, for j = 1..size-1
|
||
for (auto i = 1u; i < block_size; i++) {
|
||
for (auto j = 1; j < block_size; j++)
|
||
predicted_sample_at(i, j) = predicted_sample_at(i - 1, j - 1);
|
||
}
|
||
break;
|
||
case PredictionMode::D153Pred:
|
||
// Otherwise if mode is equal to D153_PRED, the following applies:
|
||
// 1. pred[ 0 ][ 0 ] = Round2( leftCol[ 0 ] + aboveRow[ -1 ], 1 )
|
||
predicted_sample_at(0, 0) = rounded_right_shift(left_column[0] + above_row_at(-1), 1);
|
||
// 2. pred[ i ][ 0 ] = Round2( leftCol[ i - 1] + leftCol[ i ], 1 ) for i = 1..size-1
|
||
for (auto i = 1u; i < block_size; i++)
|
||
predicted_sample_at(i, 0) = rounded_right_shift(left_column[i - 1] + left_column[i], 1);
|
||
// 3. pred[ 0 ][ 1 ] = Round2( leftCol[ 0 ] + 2 * aboveRow[ -1 ] + aboveRow[ 0 ], 2 )
|
||
predicted_sample_at(0, 1) = rounded_right_shift(left_column[0] + 2 * above_row_at(-1) + above_row_at(0), 2);
|
||
// 4. pred[ 1 ][ 1 ] = Round2( aboveRow[ -1 ] + 2 * leftCol [ 0 ] + leftCol [ 1 ], 2 )
|
||
predicted_sample_at(1, 1) = rounded_right_shift(above_row_at(-1) + 2 * left_column[0] + left_column[1], 2);
|
||
// 5. pred[ i ][ 1 ] = Round2( leftCol[ i - 2 ] + 2 * leftCol[ i - 1 ] + leftCol[ i ], 2 ) for i = 2..size-1
|
||
for (auto i = 2u; i < block_size; i++)
|
||
predicted_sample_at(i, 1) = rounded_right_shift(left_column[i - 2] + 2 * left_column[i - 1] + left_column[i], 2);
|
||
// 6. pred[ 0 ][ j ] = Round2( aboveRow[ j - 3 ] + 2 * aboveRow[ j - 2 ] + aboveRow[ j - 1 ], 2 ) for j = 2..size-1
|
||
for (auto j = 2; j < block_size; j++)
|
||
predicted_sample_at(0, j) = rounded_right_shift(above_row_at(j - 3) + 2 * above_row_at(j - 2) + above_row_at(j - 1), 2);
|
||
// 7. pred[ i ][ j ] = pred[ i - 1 ][ j - 2 ] for i = 1..size-1, for j = 2..size-1
|
||
for (auto i = 1u; i < block_size; i++) {
|
||
for (auto j = 2u; j < block_size; j++)
|
||
predicted_sample_at(i, j) = predicted_sample_at(i - 1, j - 2);
|
||
}
|
||
break;
|
||
case PredictionMode::TmPred:
|
||
// Otherwise if mode is equal to TM_PRED,
|
||
// pred[ i ][ j ] is set equal to Clip1( aboveRow[ j ] + leftCol[ i ] - aboveRow[ -1 ] )
|
||
// for i = 0..size-1, for j = 0..size-1.
|
||
for (auto i = 0u; i < block_size; i++) {
|
||
for (auto j = 0u; j < block_size; j++)
|
||
predicted_sample_at(i, j) = clip_1(block_context.frame_context.color_config.bit_depth, above_row_at(j) + left_column[i] - above_row_at(-1));
|
||
}
|
||
break;
|
||
case PredictionMode::DcPred: {
|
||
Intermediate average = 0;
|
||
|
||
if (have_left && have_above) {
|
||
// Otherwise if mode is equal to DC_PRED and haveLeft is equal to 1 and haveAbove is equal to 1,
|
||
// The variable avg (the average of the samples in union of aboveRow and leftCol)
|
||
// is specified as follows:
|
||
// sum = 0
|
||
// for ( k = 0; k < size; k++ ) {
|
||
// sum += leftCol[ k ]
|
||
// sum += aboveRow[ k ]
|
||
// }
|
||
// avg = (sum + size) >> (log2Size + 1)
|
||
Intermediate sum = 0;
|
||
for (auto k = 0u; k < block_size; k++) {
|
||
sum += left_column[k];
|
||
sum += above_row_at(k);
|
||
}
|
||
average = (sum + block_size) >> (log2_of_block_size + 1);
|
||
} else if (have_left && !have_above) {
|
||
// Otherwise if mode is equal to DC_PRED and haveLeft is equal to 1 and haveAbove is equal to 0,
|
||
// The variable leftAvg is specified as follows:
|
||
// sum = 0
|
||
// for ( k = 0; k < size; k++ ) {
|
||
// sum += leftCol[ k ]
|
||
// }
|
||
// leftAvg = (sum + (1 << (log2Size - 1) ) ) >> log2Size
|
||
Intermediate sum = 0;
|
||
for (auto k = 0u; k < block_size; k++)
|
||
sum += left_column[k];
|
||
average = (sum + (1 << (log2_of_block_size - 1))) >> log2_of_block_size;
|
||
} else if (!have_left && have_above) {
|
||
// Otherwise if mode is equal to DC_PRED and haveLeft is equal to 0 and haveAbove is equal to 1,
|
||
// The variable aboveAvg is specified as follows:
|
||
// sum = 0
|
||
// for ( k = 0; k < size; k++ ) {
|
||
// sum += aboveRow[ k ]
|
||
// }
|
||
// aboveAvg = (sum + (1 << (log2Size - 1) ) ) >> log2Size
|
||
Intermediate sum = 0;
|
||
for (auto k = 0u; k < block_size; k++)
|
||
sum += above_row_at(k);
|
||
average = (sum + (1 << (log2_of_block_size - 1))) >> log2_of_block_size;
|
||
} else {
|
||
// Otherwise (mode is DC_PRED),
|
||
// pred[ i ][ j ] is set equal to 1<<(BitDepth - 1) with i = 0..size-1 and j = 0..size-1.
|
||
average = 1 << (block_context.frame_context.color_config.bit_depth - 1);
|
||
}
|
||
|
||
// pred[ i ][ j ] is set equal to avg with i = 0..size-1 and j = 0..size-1.
|
||
for (auto i = 0u; i < block_size; i++) {
|
||
for (auto j = 0u; j < block_size; j++)
|
||
predicted_sample_at(i, j) = average;
|
||
}
|
||
break;
|
||
}
|
||
default:
|
||
dbgln("Unknown prediction mode {}", static_cast<u8>(mode));
|
||
VERIFY_NOT_REACHED();
|
||
}
|
||
|
||
// The current frame is updated as follows:
|
||
// − CurrFrame[ plane ][ y + i ][ x + j ] is set equal to pred[ i ][ j ] for i = 0..size-1 and j = 0..size-1.
|
||
auto width_in_frame_buffer = min(static_cast<u32>(block_size), max_x - x + 1);
|
||
auto height_in_frame_buffer = min(static_cast<u32>(block_size), max_y - y + 1);
|
||
|
||
for (auto i = 0u; i < height_in_frame_buffer; i++) {
|
||
for (auto j = 0u; j < width_in_frame_buffer; j++)
|
||
frame_buffer_at(y + i, x + j) = predicted_sample_at(i, j);
|
||
}
|
||
|
||
return {};
|
||
}
|
||
|
||
MotionVector Decoder::select_motion_vector(u8 plane, BlockContext const& block_context, ReferenceIndex reference_index, u32 block_index)
|
||
{
|
||
// The inputs to this process are:
|
||
// − a variable plane specifying which plane is being predicted,
|
||
// − a variable refList specifying that we should select the motion vector from BlockMvs[ refList ],
|
||
// − a variable blockIdx, specifying how much of the block has already been predicted in units of 4x4 samples.
|
||
// The output of this process is a 2 element array called mv containing the motion vector for this block.
|
||
|
||
// The purpose of this process is to find the motion vector for this block. Motion vectors are specified for each
|
||
// luma block, but a chroma block may cover more than one luma block due to subsampling. In this case, an
|
||
// average motion vector is constructed for the chroma block.
|
||
|
||
// The functions round_mv_comp_q2 and round_mv_comp_q4 perform division with rounding to the nearest
|
||
// integer and are specified as:
|
||
auto round_mv_comp_q2 = [&](MotionVector in) {
|
||
// return (value < 0 ? value - 1 : value + 1) / 2
|
||
return MotionVector {
|
||
(in.row() < 0 ? in.row() - 1 : in.row() + 1) / 2,
|
||
(in.column() < 0 ? in.column() - 1 : in.column() + 1) / 2
|
||
};
|
||
};
|
||
auto round_mv_comp_q4 = [&](MotionVector in) {
|
||
// return (value < 0 ? value - 2 : value + 2) / 4
|
||
return MotionVector {
|
||
(in.row() < 0 ? in.row() - 2 : in.row() + 2) / 4,
|
||
(in.column() < 0 ? in.column() - 2 : in.column() + 2) / 4
|
||
};
|
||
};
|
||
|
||
auto vectors = block_context.sub_block_motion_vectors;
|
||
|
||
// The motion vector array mv is derived as follows:
|
||
// − If plane is equal to 0, or MiSize is greater than or equal to BLOCK_8X8, mv is set equal to
|
||
// BlockMvs[ refList ][ blockIdx ].
|
||
if (plane == 0 || block_context.size >= Block_8x8)
|
||
return vectors[block_index][reference_index];
|
||
// − Otherwise, if subsampling_x is equal to 0 and subsampling_y is equal to 0, mv is set equal to
|
||
// BlockMvs[ refList ][ blockIdx ].
|
||
if (!block_context.frame_context.color_config.subsampling_x && !block_context.frame_context.color_config.subsampling_y)
|
||
return vectors[block_index][reference_index];
|
||
// − Otherwise, if subsampling_x is equal to 0 and subsampling_y is equal to 1, mv[ comp ] is set equal to
|
||
// round_mv_comp_q2( BlockMvs[ refList ][ blockIdx ][ comp ] + BlockMvs[ refList ][ blockIdx + 2 ][ comp ] )
|
||
// for comp = 0..1.
|
||
if (!block_context.frame_context.color_config.subsampling_x && block_context.frame_context.color_config.subsampling_y)
|
||
return round_mv_comp_q2(vectors[block_index][reference_index] + vectors[block_index + 2][reference_index]);
|
||
// − Otherwise, if subsampling_x is equal to 1 and subsampling_y is equal to 0, mv[ comp ] is set equal to
|
||
// round_mv_comp_q2( BlockMvs[ refList ][ blockIdx ][ comp ] + BlockMvs[ refList ][ blockIdx + 1 ][ comp ] )
|
||
// for comp = 0..1.
|
||
if (block_context.frame_context.color_config.subsampling_x && !block_context.frame_context.color_config.subsampling_y)
|
||
return round_mv_comp_q2(vectors[block_index][reference_index] + vectors[block_index + 1][reference_index]);
|
||
// − Otherwise, (subsampling_x is equal to 1 and subsampling_y is equal to 1), mv[ comp ] is set equal to
|
||
// round_mv_comp_q4( BlockMvs[ refList ][ 0 ][ comp ] + BlockMvs[ refList ][ 1 ][ comp ] +
|
||
// BlockMvs[ refList ][ 2 ][ comp ] + BlockMvs[ refList ][ 3 ][ comp ] ) for comp = 0..1.
|
||
VERIFY(block_context.frame_context.color_config.subsampling_x && block_context.frame_context.color_config.subsampling_y);
|
||
return round_mv_comp_q4(vectors[0][reference_index] + vectors[1][reference_index]
|
||
+ vectors[2][reference_index] + vectors[3][reference_index]);
|
||
}
|
||
|
||
MotionVector Decoder::clamp_motion_vector(u8 plane, BlockContext const& block_context, u32 block_row, u32 block_column, MotionVector vector)
|
||
{
|
||
// FIXME: This function is named very similarly to Parser::clamp_mv. Rename one or the other?
|
||
|
||
// The purpose of this process is to change the motion vector into the appropriate precision for the current plane
|
||
// and to clamp motion vectors that go too far off the edge of the frame.
|
||
// The variables sx and sy are set equal to the subsampling for the current plane as follows:
|
||
// − If plane is equal to 0, sx is set equal to 0 and sy is set equal to 0.
|
||
// − Otherwise, sx is set equal to subsampling_x and sy is set equal to subsampling_y.
|
||
bool subsampling_x = plane > 0 ? block_context.frame_context.color_config.subsampling_x : false;
|
||
bool subsampling_y = plane > 0 ? block_context.frame_context.color_config.subsampling_y : false;
|
||
|
||
// The output array clampedMv is specified by the following steps:
|
||
i32 blocks_high = num_8x8_blocks_high_lookup[block_context.size];
|
||
// Casts must be done here to prevent subtraction underflow from wrapping the values.
|
||
i32 mb_to_top_edge = -(static_cast<i32>(block_row * MI_SIZE) * 16) >> subsampling_y;
|
||
i32 mb_to_bottom_edge = (((static_cast<i32>(block_context.frame_context.rows()) - blocks_high - static_cast<i32>(block_row)) * MI_SIZE) * 16) >> subsampling_y;
|
||
|
||
i32 blocks_wide = num_8x8_blocks_wide_lookup[block_context.size];
|
||
i32 mb_to_left_edge = -(static_cast<i32>(block_column * MI_SIZE) * 16) >> subsampling_x;
|
||
i32 mb_to_right_edge = (((static_cast<i32>(block_context.frame_context.columns()) - blocks_wide - static_cast<i32>(block_column)) * MI_SIZE) * 16) >> subsampling_x;
|
||
|
||
i32 subpel_left = (INTERP_EXTEND + ((blocks_wide * MI_SIZE) >> subsampling_x)) << SUBPEL_BITS;
|
||
i32 subpel_right = subpel_left - SUBPEL_SHIFTS;
|
||
i32 subpel_top = (INTERP_EXTEND + ((blocks_high * MI_SIZE) >> subsampling_y)) << SUBPEL_BITS;
|
||
i32 subpel_bottom = subpel_top - SUBPEL_SHIFTS;
|
||
return {
|
||
clip_3(mb_to_top_edge - subpel_top, mb_to_bottom_edge + subpel_bottom, (2 * vector.row()) >> subsampling_y),
|
||
clip_3(mb_to_left_edge - subpel_left, mb_to_right_edge + subpel_right, (2 * vector.column()) >> subsampling_x)
|
||
};
|
||
}
|
||
|
||
static constexpr i32 maximum_scaled_step = 80;
|
||
|
||
DecoderErrorOr<void> Decoder::prepare_referenced_frame(Gfx::Size<u32> frame_size, u8 reference_frame_index)
|
||
{
|
||
ReferenceFrame& reference_frame = m_parser->m_reference_frames[reference_frame_index];
|
||
|
||
// 8.5.2.3 Motion vector scaling process
|
||
// The inputs to this process are:
|
||
// − a variable plane specifying which plane is being predicted,
|
||
// − a variable refList specifying that we should scale to match reference frame ref_frame[ refList ],
|
||
// − variables x and y specifying the location of the top left sample in the CurrFrame[ plane ] array of the region
|
||
// to be predicted,
|
||
// − a variable clampedMv specifying the clamped motion vector.
|
||
// The outputs of this process are the variables startX and startY giving the reference block location in units of
|
||
// 1/16 th of a sample, and variables xStep and yStep giving the step size in units of 1/16 th of a sample.
|
||
// This process is responsible for computing the sampling locations in the reference frame based on the motion
|
||
// vector. The sampling locations are also adjusted to compensate for any difference in the size of the reference
|
||
// frame compared to the current frame.
|
||
|
||
// It is a requirement of bitstream conformance that all the following conditions are satisfied:
|
||
// − 2 * FrameWidth >= RefFrameWidth[ refIdx ]
|
||
// − 2 * FrameHeight >= RefFrameHeight[ refIdx ]
|
||
// − FrameWidth <= 16 * RefFrameWidth[ refIdx ]
|
||
// − FrameHeight <= 16 * RefFrameHeight[ refIdx ]
|
||
if (!reference_frame.is_valid())
|
||
return DecoderError::format(DecoderErrorCategory::Corrupted, "Attempted to use reference frame {} that has not been saved", reference_frame_index);
|
||
auto double_frame_size = frame_size.scaled_by(2);
|
||
if (double_frame_size.width() < reference_frame.size.width() || double_frame_size.height() < reference_frame.size.height())
|
||
return DecoderError::format(DecoderErrorCategory::Corrupted, "Inter frame size is too small relative to reference frame {}", reference_frame_index);
|
||
if (!reference_frame.size.scaled_by(16).contains(frame_size))
|
||
return DecoderError::format(DecoderErrorCategory::Corrupted, "Inter frame size is too large relative to reference frame {}", reference_frame_index);
|
||
|
||
// FIXME: Convert all the operations in this function to vector operations supported by
|
||
// MotionVector.
|
||
|
||
// A variable xScale is set equal to (RefFrameWidth[ refIdx ] << REF_SCALE_SHIFT) / FrameWidth.
|
||
// A variable yScale is set equal to (RefFrameHeight[ refIdx ] << REF_SCALE_SHIFT) / FrameHeight.
|
||
// (xScale and yScale specify the size of the reference frame relative to the current frame in units where 16 is
|
||
// equivalent to the reference frame having the same size.)
|
||
// NOTE: This spec note above seems to be incorrect. The 1:1 scale value would be 16,384.
|
||
i32 x_scale = (reference_frame.size.width() << REF_SCALE_SHIFT) / frame_size.width();
|
||
i32 y_scale = (reference_frame.size.height() << REF_SCALE_SHIFT) / frame_size.height();
|
||
|
||
// The output variable stepX is set equal to (16 * xScale) >> REF_SCALE_SHIFT.
|
||
// The output variable stepY is set equal to (16 * yScale) >> REF_SCALE_SHIFT.
|
||
i32 scaled_step_x = (16 * x_scale) >> REF_SCALE_SHIFT;
|
||
i32 scaled_step_y = (16 * y_scale) >> REF_SCALE_SHIFT;
|
||
|
||
// 5. The block inter prediction process in section 8.5.2.4 is invoked with plane, refList, startX, startY, stepX,
|
||
// stepY, w, h as inputs and the output is assigned to the 2D array preds[ refList ].
|
||
|
||
// 8.5.2.4 Block inter prediction process
|
||
// The inputs to this process are:
|
||
// − a variable plane,
|
||
// − a variable refList specifying that we should predict from ref_frame[ refList ],
|
||
// − variables x and y giving the block location in units of 1/16 th of a sample,
|
||
// − variables xStep and yStep giving the step size in units of 1/16 th of a sample. (These will be at most equal
|
||
// to 80 due to the restrictions on scaling between reference frames.)
|
||
VERIFY(scaled_step_x <= maximum_scaled_step && scaled_step_y <= maximum_scaled_step);
|
||
// − variables w and h giving the width and height of the block in units of samples
|
||
// The output from this process is the 2D array named pred containing inter predicted samples.
|
||
|
||
reference_frame.x_scale = x_scale;
|
||
reference_frame.y_scale = x_scale;
|
||
reference_frame.scaled_step_x = scaled_step_x;
|
||
reference_frame.scaled_step_y = scaled_step_y;
|
||
|
||
return {};
|
||
}
|
||
|
||
DecoderErrorOr<void> Decoder::predict_inter_block(u8 plane, BlockContext const& block_context, ReferenceIndex reference_index, u32 block_row, u32 block_column, u32 x, u32 y, u32 width, u32 height, u32 block_index, Span<u16> block_buffer)
|
||
{
|
||
VERIFY(width <= maximum_block_dimensions && height <= maximum_block_dimensions);
|
||
// 2. The motion vector selection process in section 8.5.2.1 is invoked with plane, refList, blockIdx as inputs
|
||
// and the output being the motion vector mv.
|
||
auto motion_vector = select_motion_vector(plane, block_context, reference_index, block_index);
|
||
|
||
// 3. The motion vector clamping process in section 8.5.2.2 is invoked with plane, mv as inputs and the output
|
||
// being the clamped motion vector clampedMv
|
||
auto clamped_vector = clamp_motion_vector(plane, block_context, block_row, block_column, motion_vector);
|
||
|
||
// 4. The motion vector scaling process in section 8.5.2.3 is invoked with plane, refList, x, y, clampedMv as
|
||
// inputs and the output being the initial location startX, startY, and the step sizes stepX, stepY.
|
||
|
||
// 8.5.2.3 Motion vector scaling process
|
||
// The inputs to this process are:
|
||
// − a variable plane specifying which plane is being predicted,
|
||
// − a variable refList specifying that we should scale to match reference frame ref_frame[ refList ],
|
||
// − variables x and y specifying the location of the top left sample in the CurrFrame[ plane ] array of the region
|
||
// to be predicted,
|
||
// − a variable clampedMv specifying the clamped motion vector.
|
||
// The outputs of this process are the variables startX and startY giving the reference block location in units of
|
||
// 1/16 th of a sample, and variables xStep and yStep giving the step size in units of 1/16 th of a sample.
|
||
// This process is responsible for computing the sampling locations in the reference frame based on the motion
|
||
// vector. The sampling locations are also adjusted to compensate for any difference in the size of the reference
|
||
// frame compared to the current frame.
|
||
|
||
// NOTE: Some of this is done in advance by Decoder::prepare_referenced_frame().
|
||
|
||
// A variable refIdx specifying which reference frame is being used is set equal to
|
||
// ref_frame_idx[ ref_frame[ refList ] - LAST_FRAME ].
|
||
auto reference_frame_index = block_context.frame_context.reference_frame_indices[block_context.reference_frame_types[reference_index] - ReferenceFrameType::LastFrame];
|
||
auto const& reference_frame = m_parser->m_reference_frames[reference_frame_index];
|
||
|
||
// Scale values range from 8192 to 262144.
|
||
// 16384 = 1:1, higher values indicate the reference frame is larger than the current frame.
|
||
auto x_scale = reference_frame.x_scale;
|
||
auto y_scale = reference_frame.y_scale;
|
||
|
||
// The amount of subpixels between each sample of this block. Non-16 values will cause the output to be scaled.
|
||
auto scaled_step_x = reference_frame.scaled_step_x;
|
||
auto scaled_step_y = reference_frame.scaled_step_y;
|
||
|
||
// The variable baseX is set equal to (x * xScale) >> REF_SCALE_SHIFT.
|
||
// The variable baseY is set equal to (y * yScale) >> REF_SCALE_SHIFT.
|
||
// (baseX and baseY specify the location of the block in the reference frame if a zero motion vector is used).
|
||
i32 base_x = (x * x_scale) >> REF_SCALE_SHIFT;
|
||
i32 base_y = (y * y_scale) >> REF_SCALE_SHIFT;
|
||
|
||
// The variable lumaX is set equal to (plane > 0) ? x << subsampling_x : x.
|
||
// The variable lumaY is set equal to (plane > 0) ? y << subsampling_y : y.
|
||
// (lumaX and lumaY specify the location of the block to be predicted in the current frame in units of luma
|
||
// samples.)
|
||
bool subsampling_x = plane > 0 ? block_context.frame_context.color_config.subsampling_x : false;
|
||
bool subsampling_y = plane > 0 ? block_context.frame_context.color_config.subsampling_y : false;
|
||
i32 luma_x = x << subsampling_x;
|
||
i32 luma_y = y << subsampling_y;
|
||
|
||
// The variable fracX is set equal to ( (16 * lumaX * xScale) >> REF_SCALE_SHIFT) & SUBPEL_MASK.
|
||
// The variable fracY is set equal to ( (16 * lumaY * yScale) >> REF_SCALE_SHIFT) & SUBPEL_MASK.
|
||
i32 frac_x = ((16 * luma_x * x_scale) >> REF_SCALE_SHIFT) & SUBPEL_MASK;
|
||
i32 frac_y = ((16 * luma_y * y_scale) >> REF_SCALE_SHIFT) & SUBPEL_MASK;
|
||
|
||
// The variable dX is set equal to ( (clampedMv[ 1 ] * xScale) >> REF_SCALE_SHIFT) + fracX.
|
||
// The variable dY is set equal to ( (clampedMv[ 0 ] * yScale) >> REF_SCALE_SHIFT) + fracY.
|
||
// (dX and dY specify a scaled motion vector.)
|
||
i32 scaled_vector_x = ((clamped_vector.column() * x_scale) >> REF_SCALE_SHIFT) + frac_x;
|
||
i32 scaled_vector_y = ((clamped_vector.row() * y_scale) >> REF_SCALE_SHIFT) + frac_y;
|
||
|
||
// The output variable startX is set equal to (baseX << SUBPEL_BITS) + dX.
|
||
// The output variable startY is set equal to (baseY << SUBPEL_BITS) + dY.
|
||
i32 offset_scaled_block_x = (base_x << SUBPEL_BITS) + scaled_vector_x;
|
||
i32 offset_scaled_block_y = (base_y << SUBPEL_BITS) + scaled_vector_y;
|
||
|
||
// A variable ref specifying the reference frame contents is set equal to FrameStore[ refIdx ].
|
||
auto& reference_frame_buffer = reference_frame.frame_planes[plane];
|
||
auto reference_frame_width = y_size_to_uv_size(subsampling_x, reference_frame.size.width()) + MV_BORDER * 2;
|
||
|
||
// The variable lastX is set equal to ( (RefFrameWidth[ refIdx ] + subX) >> subX) - 1.
|
||
// The variable lastY is set equal to ( (RefFrameHeight[ refIdx ] + subY) >> subY) - 1.
|
||
// (lastX and lastY specify the coordinates of the bottom right sample of the reference plane.)
|
||
// Ad-hoc: These variables are not needed, since the reference frame is expanded to contain the samples that
|
||
// may be referenced by motion vectors on the edge of the frame.
|
||
|
||
// The sub-sample interpolation is effected via two one-dimensional convolutions. First a horizontal filter is used
|
||
// to build up a temporary array, and then this array is vertically filtered to obtain the final prediction. The
|
||
// fractional parts of the motion vectors determine the filtering process. If the fractional part is zero, then the
|
||
// filtering is equivalent to a straight sample copy.
|
||
// The filtering is applied as follows:
|
||
|
||
constexpr auto sample_offset = 3;
|
||
|
||
auto subpixel_row_from_reference_row = [offset_scaled_block_y](u32 row) {
|
||
return (offset_scaled_block_y >> SUBPEL_BITS) + static_cast<i32>(row);
|
||
};
|
||
auto reference_index_for_row = [reference_frame_width](i32 row) {
|
||
return static_cast<size_t>(MV_BORDER + row) * reference_frame_width;
|
||
};
|
||
|
||
// The variable intermediateHeight specifying the height required for the intermediate array is set equal to (((h -
|
||
// 1) * yStep + 15) >> 4) + 8.
|
||
static constexpr auto maximum_intermediate_height = (((maximum_block_dimensions - 1) * maximum_scaled_step + 15) >> 4) + 8;
|
||
auto const intermediate_height = (((height - 1) * scaled_step_y + 15) >> 4) + 8;
|
||
VERIFY(intermediate_height <= maximum_intermediate_height);
|
||
// Check our reference frame bounds before starting the loop.
|
||
auto const last_possible_reference_index = reference_index_for_row(subpixel_row_from_reference_row(intermediate_height - sample_offset));
|
||
VERIFY(reference_frame_buffer.size() >= last_possible_reference_index);
|
||
|
||
VERIFY(block_buffer.size() >= static_cast<size_t>(width) * height);
|
||
|
||
auto const reference_block_x = MV_BORDER + (offset_scaled_block_x >> SUBPEL_BITS);
|
||
auto const reference_block_y = MV_BORDER + (offset_scaled_block_y >> SUBPEL_BITS);
|
||
auto const reference_subpixel_x = offset_scaled_block_x & SUBPEL_MASK;
|
||
auto const reference_subpixel_y = offset_scaled_block_y & SUBPEL_MASK;
|
||
|
||
// OPTIMIZATION: If the fractional part of a component of the motion vector is 0, we want to do a fast path
|
||
// skipping one or both of the convolutions.
|
||
bool const copy_x = reference_subpixel_x == 0;
|
||
bool const copy_y = reference_subpixel_y == 0;
|
||
bool const unscaled_x = scaled_step_x == 16;
|
||
bool const unscaled_y = scaled_step_y == 16;
|
||
|
||
// The array intermediate is specified as follows:
|
||
// Note: Height is specified by `intermediate_height`, width is specified by `width`
|
||
Array<u16, maximum_intermediate_height * maximum_block_dimensions> intermediate_buffer;
|
||
auto const bit_depth = block_context.frame_context.color_config.bit_depth;
|
||
auto const* reference_start = reference_frame_buffer.data() + reference_block_y * reference_frame_width + reference_block_x;
|
||
|
||
// FIXME: We are using 16-bit products to vectorize the filter loops, but when filtering in a high bit-depth video, they will truncate.
|
||
// Instead of hardcoding them, we should have the bit depth as a template parameter, and the accumulators can select a size based
|
||
// on whether the bit depth > 8.
|
||
// Note that we only get a benefit from this on the default CPU target. If we enable AVX2 here, we may want to specialize the
|
||
// function for the CPU target and remove the cast to i16 so that it doesn't have to truncate on AVX2, where it can do the full
|
||
// unrolled 32-bit product loops in one vector.
|
||
|
||
if (unscaled_x && unscaled_y && bit_depth == 8) {
|
||
if (copy_x && copy_y) {
|
||
// We can memcpy here to avoid doing any real work.
|
||
auto const* reference_scan_line = &reference_frame_buffer[reference_block_y * reference_frame_width + reference_block_x];
|
||
auto* destination_scan_line = block_buffer.data();
|
||
|
||
for (auto row = 0u; row < height; row++) {
|
||
memcpy(destination_scan_line, reference_scan_line, width * sizeof(*destination_scan_line));
|
||
reference_scan_line += reference_frame_width;
|
||
destination_scan_line += width;
|
||
}
|
||
|
||
return {};
|
||
}
|
||
|
||
auto horizontal_convolution_unscaled = [](auto bit_depth, auto* destination, auto width, auto height, auto const* source, auto source_stride, auto filter, auto subpixel_x) {
|
||
source -= sample_offset;
|
||
auto const source_end_skip = source_stride - width;
|
||
|
||
for (auto row = 0u; row < height; row++) {
|
||
for (auto column = 0u; column < width; column++) {
|
||
i32 accumulated_samples = 0;
|
||
for (auto t = 0; t < 8; t++) {
|
||
auto sample = source[t];
|
||
accumulated_samples += static_cast<i16>(subpel_filters[filter][subpixel_x][t] * sample);
|
||
}
|
||
|
||
*destination = clip_1(bit_depth, rounded_right_shift(accumulated_samples, 7));
|
||
source++;
|
||
destination++;
|
||
}
|
||
source += source_end_skip;
|
||
}
|
||
};
|
||
|
||
if (copy_y) {
|
||
horizontal_convolution_unscaled(bit_depth, block_buffer.data(), width, height, reference_start, reference_frame_width, block_context.interpolation_filter, reference_subpixel_x);
|
||
return {};
|
||
}
|
||
|
||
auto vertical_convolution_unscaled = [](auto bit_depth, auto* destination, auto width, auto height, auto const* source, auto source_stride, auto filter, auto subpixel_y) {
|
||
auto const source_end_skip = source_stride - width;
|
||
|
||
for (auto row = 0u; row < height; row++) {
|
||
for (auto column = 0u; column < width; column++) {
|
||
auto const* scan_column = source;
|
||
i32 accumulated_samples = 0;
|
||
for (auto t = 0; t < 8; t++) {
|
||
auto sample = *scan_column;
|
||
accumulated_samples += static_cast<i16>(subpel_filters[filter][subpixel_y][t] * sample);
|
||
scan_column += source_stride;
|
||
}
|
||
*destination = clip_1(bit_depth, rounded_right_shift(accumulated_samples, 7));
|
||
source++;
|
||
destination++;
|
||
}
|
||
source += source_end_skip;
|
||
}
|
||
};
|
||
|
||
if (copy_x) {
|
||
vertical_convolution_unscaled(bit_depth, block_buffer.data(), width, height, reference_start - (sample_offset * reference_frame_width), reference_frame_width, block_context.interpolation_filter, reference_subpixel_y);
|
||
return {};
|
||
}
|
||
|
||
horizontal_convolution_unscaled(bit_depth, intermediate_buffer.data(), width, intermediate_height, reference_start - (sample_offset * reference_frame_width), reference_frame_width, block_context.interpolation_filter, reference_subpixel_x);
|
||
vertical_convolution_unscaled(bit_depth, block_buffer.data(), width, height, intermediate_buffer.data(), width, block_context.interpolation_filter, reference_subpixel_y);
|
||
return {};
|
||
}
|
||
|
||
// NOTE: Accumulators below are 32-bit to allow high bit-depth videos to decode without overflows.
|
||
// These should be changed when the accumulators above are.
|
||
|
||
auto horizontal_convolution_scaled = [](auto bit_depth, auto* destination, auto width, auto height, auto const* source, auto source_stride, auto filter, auto subpixel_x, auto scale_x) {
|
||
source -= sample_offset;
|
||
|
||
for (auto row = 0u; row < height; row++) {
|
||
auto scan_subpixel = subpixel_x;
|
||
for (auto column = 0u; column < width; column++) {
|
||
auto const* scan_line = source + (scan_subpixel >> 4);
|
||
i32 accumulated_samples = 0;
|
||
for (auto t = 0; t < 8; t++) {
|
||
auto sample = scan_line[t];
|
||
accumulated_samples += subpel_filters[filter][scan_subpixel & SUBPEL_MASK][t] * sample;
|
||
}
|
||
|
||
*destination = clip_1(bit_depth, rounded_right_shift(accumulated_samples, 7));
|
||
destination++;
|
||
scan_subpixel += scale_x;
|
||
}
|
||
source += source_stride;
|
||
}
|
||
};
|
||
|
||
auto vertical_convolution_scaled = [](auto bit_depth, auto* destination, auto width, auto height, auto const* source, auto source_stride, auto filter, auto subpixel_y, auto scale_y) {
|
||
for (auto row = 0u; row < height; row++) {
|
||
auto const* source_column_base = source + (subpixel_y >> SUBPEL_BITS) * source_stride;
|
||
|
||
for (auto column = 0u; column < width; column++) {
|
||
auto const* scan_column = source_column_base + column;
|
||
i32 accumulated_samples = 0;
|
||
for (auto t = 0; t < 8; t++) {
|
||
auto sample = *scan_column;
|
||
accumulated_samples += subpel_filters[filter][subpixel_y & SUBPEL_MASK][t] * sample;
|
||
scan_column += source_stride;
|
||
}
|
||
|
||
*destination = clip_1(bit_depth, rounded_right_shift(accumulated_samples, 7));
|
||
destination++;
|
||
}
|
||
subpixel_y += scale_y;
|
||
}
|
||
};
|
||
|
||
horizontal_convolution_scaled(bit_depth, intermediate_buffer.data(), width, intermediate_height, reference_start - (sample_offset * reference_frame_width), reference_frame_width, block_context.interpolation_filter, offset_scaled_block_x & SUBPEL_MASK, scaled_step_x);
|
||
vertical_convolution_scaled(bit_depth, block_buffer.data(), width, height, intermediate_buffer.data(), width, block_context.interpolation_filter, reference_subpixel_y, scaled_step_y);
|
||
|
||
return {};
|
||
}
|
||
|
||
DecoderErrorOr<void> Decoder::predict_inter(u8 plane, BlockContext const& block_context, u32 x, u32 y, u32 width, u32 height, u32 block_index)
|
||
{
|
||
// The inter prediction process is invoked for inter coded blocks. When MiSize is smaller than BLOCK_8X8, the
|
||
// prediction is done with a granularity of 4x4 samples, otherwise the whole plane is predicted at the same time.
|
||
// The inputs to this process are:
|
||
// − a variable plane specifying which plane is being predicted,
|
||
// − variables x and y specifying the location of the top left sample in the CurrFrame[ plane ] array of the region
|
||
// to be predicted,
|
||
// − variables w and h specifying the width and height of the region to be predicted,
|
||
// − a variable blockIdx, specifying how much of the block has already been predicted in units of 4x4 samples.
|
||
// The outputs of this process are inter predicted samples in the current frame CurrFrame.
|
||
|
||
// The prediction arrays are formed by the following ordered steps:
|
||
// 1. The variable refList is set equal to 0.
|
||
// 2. through 5.
|
||
Array<u16, maximum_block_size> predicted_buffer;
|
||
auto predicted_span = predicted_buffer.span().trim(width * height);
|
||
TRY(predict_inter_block(plane, block_context, ReferenceIndex::Primary, block_context.row, block_context.column, x, y, width, height, block_index, predicted_span));
|
||
auto predicted_buffer_at = [&](Span<u16> buffer, u32 row, u32 column) -> u16& {
|
||
return buffer[row * width + column];
|
||
};
|
||
|
||
// 6. If isCompound is equal to 1, then the variable refList is set equal to 1 and steps 2, 3, 4 and 5 are repeated
|
||
// to form the prediction for the second reference.
|
||
// The inter predicted samples are then derived as follows:
|
||
auto& frame_buffer = get_output_buffer(plane);
|
||
VERIFY(!frame_buffer.is_empty());
|
||
auto frame_size = block_context.frame_context.decoded_size(plane > 0);
|
||
auto frame_buffer_at = [&](u32 row, u32 column) -> u16& {
|
||
return frame_buffer[row * frame_size.width() + column];
|
||
};
|
||
|
||
auto width_in_frame_buffer = min(width, frame_size.width() - x);
|
||
auto height_in_frame_buffer = min(height, frame_size.height() - y);
|
||
|
||
// The variable isCompound is set equal to ref_frame[ 1 ] > NONE.
|
||
// − If isCompound is equal to 0, CurrFrame[ plane ][ y + i ][ x + j ] is set equal to preds[ 0 ][ i ][ j ] for i = 0..h-1
|
||
// and j = 0..w-1.
|
||
if (!block_context.is_compound()) {
|
||
for (auto i = 0u; i < height_in_frame_buffer; i++) {
|
||
for (auto j = 0u; j < width_in_frame_buffer; j++)
|
||
frame_buffer_at(y + i, x + j) = predicted_buffer_at(predicted_span, i, j);
|
||
}
|
||
|
||
return {};
|
||
}
|
||
|
||
// − Otherwise, CurrFrame[ plane ][ y + i ][ x + j ] is set equal to Round2( preds[ 0 ][ i ][ j ] + preds[ 1 ][ i ][ j ], 1 )
|
||
// for i = 0..h-1 and j = 0..w-1.
|
||
Array<u16, maximum_block_size> second_predicted_buffer;
|
||
auto second_predicted_span = second_predicted_buffer.span().trim(width * height);
|
||
TRY(predict_inter_block(plane, block_context, ReferenceIndex::Secondary, block_context.row, block_context.column, x, y, width, height, block_index, second_predicted_span));
|
||
|
||
for (auto i = 0u; i < height_in_frame_buffer; i++) {
|
||
for (auto j = 0u; j < width_in_frame_buffer; j++)
|
||
frame_buffer_at(y + i, x + j) = rounded_right_shift(predicted_buffer_at(predicted_span, i, j) + predicted_buffer_at(second_predicted_span, i, j), 1);
|
||
}
|
||
|
||
return {};
|
||
}
|
||
|
||
inline u16 dc_q(u8 bit_depth, u8 b)
|
||
{
|
||
// The function dc_q( b ) is specified as dc_qlookup[ (BitDepth-8) >> 1 ][ Clip3( 0, 255, b ) ] where dc_lookup is
|
||
// defined as follows:
|
||
constexpr u16 dc_qlookup[3][256] = {
|
||
{ 4, 8, 8, 9, 10, 11, 12, 12, 13, 14, 15, 16, 17, 18, 19, 19, 20, 21, 22, 23, 24, 25, 26, 26, 27, 28, 29, 30, 31, 32, 32, 33, 34, 35, 36, 37, 38, 38, 39, 40, 41, 42, 43, 43, 44, 45, 46, 47, 48, 48, 49, 50, 51, 52, 53, 53, 54, 55, 56, 57, 57, 58, 59, 60, 61, 62, 62, 63, 64, 65, 66, 66, 67, 68, 69, 70, 70, 71, 72, 73, 74, 74, 75, 76, 77, 78, 78, 79, 80, 81, 81, 82, 83, 84, 85, 85, 87, 88, 90, 92, 93, 95, 96, 98, 99, 101, 102, 104, 105, 107, 108, 110, 111, 113, 114, 116, 117, 118, 120, 121, 123, 125, 127, 129, 131, 134, 136, 138, 140, 142, 144, 146, 148, 150, 152, 154, 156, 158, 161, 164, 166, 169, 172, 174, 177, 180, 182, 185, 187, 190, 192, 195, 199, 202, 205, 208, 211, 214, 217, 220, 223, 226, 230, 233, 237, 240, 243, 247, 250, 253, 257, 261, 265, 269, 272, 276, 280, 284, 288, 292, 296, 300, 304, 309, 313, 317, 322, 326, 330, 335, 340, 344, 349, 354, 359, 364, 369, 374, 379, 384, 389, 395, 400, 406, 411, 417, 423, 429, 435, 441, 447, 454, 461, 467, 475, 482, 489, 497, 505, 513, 522, 530, 539, 549, 559, 569, 579, 590, 602, 614, 626, 640, 654, 668, 684, 700, 717, 736, 755, 775, 796, 819, 843, 869, 896, 925, 955, 988, 1022, 1058, 1098, 1139, 1184, 1232, 1282, 1336 },
|
||
{ 4, 9, 10, 13, 15, 17, 20, 22, 25, 28, 31, 34, 37, 40, 43, 47, 50, 53, 57, 60, 64, 68, 71, 75, 78, 82, 86, 90, 93, 97, 101, 105, 109, 113, 116, 120, 124, 128, 132, 136, 140, 143, 147, 151, 155, 159, 163, 166, 170, 174, 178, 182, 185, 189, 193, 197, 200, 204, 208, 212, 215, 219, 223, 226, 230, 233, 237, 241, 244, 248, 251, 255, 259, 262, 266, 269, 273, 276, 280, 283, 287, 290, 293, 297, 300, 304, 307, 310, 314, 317, 321, 324, 327, 331, 334, 337, 343, 350, 356, 362, 369, 375, 381, 387, 394, 400, 406, 412, 418, 424, 430, 436, 442, 448, 454, 460, 466, 472, 478, 484, 490, 499, 507, 516, 525, 533, 542, 550, 559, 567, 576, 584, 592, 601, 609, 617, 625, 634, 644, 655, 666, 676, 687, 698, 708, 718, 729, 739, 749, 759, 770, 782, 795, 807, 819, 831, 844, 856, 868, 880, 891, 906, 920, 933, 947, 961, 975, 988, 1001, 1015, 1030, 1045, 1061, 1076, 1090, 1105, 1120, 1137, 1153, 1170, 1186, 1202, 1218, 1236, 1253, 1271, 1288, 1306, 1323, 1342, 1361, 1379, 1398, 1416, 1436, 1456, 1476, 1496, 1516, 1537, 1559, 1580, 1601, 1624, 1647, 1670, 1692, 1717, 1741, 1766, 1791, 1817, 1844, 1871, 1900, 1929, 1958, 1990, 2021, 2054, 2088, 2123, 2159, 2197, 2236, 2276, 2319, 2363, 2410, 2458, 2508, 2561, 2616, 2675, 2737, 2802, 2871, 2944, 3020, 3102, 3188, 3280, 3375, 3478, 3586, 3702, 3823, 3953, 4089, 4236, 4394, 4559, 4737, 4929, 5130, 5347 },
|
||
{ 4, 12, 18, 25, 33, 41, 50, 60, 70, 80, 91, 103, 115, 127, 140, 153, 166, 180, 194, 208, 222, 237, 251, 266, 281, 296, 312, 327, 343, 358, 374, 390, 405, 421, 437, 453, 469, 484, 500, 516, 532, 548, 564, 580, 596, 611, 627, 643, 659, 674, 690, 706, 721, 737, 752, 768, 783, 798, 814, 829, 844, 859, 874, 889, 904, 919, 934, 949, 964, 978, 993, 1008, 1022, 1037, 1051, 1065, 1080, 1094, 1108, 1122, 1136, 1151, 1165, 1179, 1192, 1206, 1220, 1234, 1248, 1261, 1275, 1288, 1302, 1315, 1329, 1342, 1368, 1393, 1419, 1444, 1469, 1494, 1519, 1544, 1569, 1594, 1618, 1643, 1668, 1692, 1717, 1741, 1765, 1789, 1814, 1838, 1862, 1885, 1909, 1933, 1957, 1992, 2027, 2061, 2096, 2130, 2165, 2199, 2233, 2267, 2300, 2334, 2367, 2400, 2434, 2467, 2499, 2532, 2575, 2618, 2661, 2704, 2746, 2788, 2830, 2872, 2913, 2954, 2995, 3036, 3076, 3127, 3177, 3226, 3275, 3324, 3373, 3421, 3469, 3517, 3565, 3621, 3677, 3733, 3788, 3843, 3897, 3951, 4005, 4058, 4119, 4181, 4241, 4301, 4361, 4420, 4479, 4546, 4612, 4677, 4742, 4807, 4871, 4942, 5013, 5083, 5153, 5222, 5291, 5367, 5442, 5517, 5591, 5665, 5745, 5825, 5905, 5984, 6063, 6149, 6234, 6319, 6404, 6495, 6587, 6678, 6769, 6867, 6966, 7064, 7163, 7269, 7376, 7483, 7599, 7715, 7832, 7958, 8085, 8214, 8352, 8492, 8635, 8788, 8945, 9104, 9275, 9450, 9639, 9832, 10031, 10245, 10465, 10702, 10946, 11210, 11482, 11776, 12081, 12409, 12750, 13118, 13501, 13913, 14343, 14807, 15290, 15812, 16356, 16943, 17575, 18237, 18949, 19718, 20521, 21387 }
|
||
};
|
||
|
||
return dc_qlookup[(bit_depth - 8) >> 1][clip_3<u8>(0, 255, b)];
|
||
}
|
||
|
||
inline u16 ac_q(u8 bit_depth, u8 b)
|
||
{
|
||
// The function ac_q( b ) is specified as ac_qlookup[ (BitDepth-8) >> 1 ][ Clip3( 0, 255, b ) ] where ac_lookup is
|
||
// defined as follows:
|
||
constexpr u16 ac_qlookup[3][256] = {
|
||
{ 4, 8, 9, 10, 11, 12, 13, 14, 15, 16, 17, 18, 19, 20, 21, 22, 23, 24, 25, 26, 27, 28, 29, 30, 31, 32, 33, 34, 35, 36, 37, 38, 39, 40, 41, 42, 43, 44, 45, 46, 47, 48, 49, 50, 51, 52, 53, 54, 55, 56, 57, 58, 59, 60, 61, 62, 63, 64, 65, 66, 67, 68, 69, 70, 71, 72, 73, 74, 75, 76, 77, 78, 79, 80, 81, 82, 83, 84, 85, 86, 87, 88, 89, 90, 91, 92, 93, 94, 95, 96, 97, 98, 99, 100, 101, 102, 104, 106, 108, 110, 112, 114, 116, 118, 120, 122, 124, 126, 128, 130, 132, 134, 136, 138, 140, 142, 144, 146, 148, 150, 152, 155, 158, 161, 164, 167, 170, 173, 176, 179, 182, 185, 188, 191, 194, 197, 200, 203, 207, 211, 215, 219, 223, 227, 231, 235, 239, 243, 247, 251, 255, 260, 265, 270, 275, 280, 285, 290, 295, 300, 305, 311, 317, 323, 329, 335, 341, 347, 353, 359, 366, 373, 380, 387, 394, 401, 408, 416, 424, 432, 440, 448, 456, 465, 474, 483, 492, 501, 510, 520, 530, 540, 550, 560, 571, 582, 593, 604, 615, 627, 639, 651, 663, 676, 689, 702, 715, 729, 743, 757, 771, 786, 801, 816, 832, 848, 864, 881, 898, 915, 933, 951, 969, 988, 1007, 1026, 1046, 1066, 1087, 1108, 1129, 1151, 1173, 1196, 1219, 1243, 1267, 1292, 1317, 1343, 1369, 1396, 1423, 1451, 1479, 1508, 1537, 1567, 1597, 1628, 1660, 1692, 1725, 1759, 1793, 1828 },
|
||
{ 4, 9, 11, 13, 16, 18, 21, 24, 27, 30, 33, 37, 40, 44, 48, 51, 55, 59, 63, 67, 71, 75, 79, 83, 88, 92, 96, 100, 105, 109, 114, 118, 122, 127, 131, 136, 140, 145, 149, 154, 158, 163, 168, 172, 177, 181, 186, 190, 195, 199, 204, 208, 213, 217, 222, 226, 231, 235, 240, 244, 249, 253, 258, 262, 267, 271, 275, 280, 284, 289, 293, 297, 302, 306, 311, 315, 319, 324, 328, 332, 337, 341, 345, 349, 354, 358, 362, 367, 371, 375, 379, 384, 388, 392, 396, 401, 409, 417, 425, 433, 441, 449, 458, 466, 474, 482, 490, 498, 506, 514, 523, 531, 539, 547, 555, 563, 571, 579, 588, 596, 604, 616, 628, 640, 652, 664, 676, 688, 700, 713, 725, 737, 749, 761, 773, 785, 797, 809, 825, 841, 857, 873, 889, 905, 922, 938, 954, 970, 986, 1002, 1018, 1038, 1058, 1078, 1098, 1118, 1138, 1158, 1178, 1198, 1218, 1242, 1266, 1290, 1314, 1338, 1362, 1386, 1411, 1435, 1463, 1491, 1519, 1547, 1575, 1603, 1631, 1663, 1695, 1727, 1759, 1791, 1823, 1859, 1895, 1931, 1967, 2003, 2039, 2079, 2119, 2159, 2199, 2239, 2283, 2327, 2371, 2415, 2459, 2507, 2555, 2603, 2651, 2703, 2755, 2807, 2859, 2915, 2971, 3027, 3083, 3143, 3203, 3263, 3327, 3391, 3455, 3523, 3591, 3659, 3731, 3803, 3876, 3952, 4028, 4104, 4184, 4264, 4348, 4432, 4516, 4604, 4692, 4784, 4876, 4972, 5068, 5168, 5268, 5372, 5476, 5584, 5692, 5804, 5916, 6032, 6148, 6268, 6388, 6512, 6640, 6768, 6900, 7036, 7172, 7312 },
|
||
{ 4, 13, 19, 27, 35, 44, 54, 64, 75, 87, 99, 112, 126, 139, 154, 168, 183, 199, 214, 230, 247, 263, 280, 297, 314, 331, 349, 366, 384, 402, 420, 438, 456, 475, 493, 511, 530, 548, 567, 586, 604, 623, 642, 660, 679, 698, 716, 735, 753, 772, 791, 809, 828, 846, 865, 884, 902, 920, 939, 957, 976, 994, 1012, 1030, 1049, 1067, 1085, 1103, 1121, 1139, 1157, 1175, 1193, 1211, 1229, 1246, 1264, 1282, 1299, 1317, 1335, 1352, 1370, 1387, 1405, 1422, 1440, 1457, 1474, 1491, 1509, 1526, 1543, 1560, 1577, 1595, 1627, 1660, 1693, 1725, 1758, 1791, 1824, 1856, 1889, 1922, 1954, 1987, 2020, 2052, 2085, 2118, 2150, 2183, 2216, 2248, 2281, 2313, 2346, 2378, 2411, 2459, 2508, 2556, 2605, 2653, 2701, 2750, 2798, 2847, 2895, 2943, 2992, 3040, 3088, 3137, 3185, 3234, 3298, 3362, 3426, 3491, 3555, 3619, 3684, 3748, 3812, 3876, 3941, 4005, 4069, 4149, 4230, 4310, 4390, 4470, 4550, 4631, 4711, 4791, 4871, 4967, 5064, 5160, 5256, 5352, 5448, 5544, 5641, 5737, 5849, 5961, 6073, 6185, 6297, 6410, 6522, 6650, 6778, 6906, 7034, 7162, 7290, 7435, 7579, 7723, 7867, 8011, 8155, 8315, 8475, 8635, 8795, 8956, 9132, 9308, 9484, 9660, 9836, 10028, 10220, 10412, 10604, 10812, 11020, 11228, 11437, 11661, 11885, 12109, 12333, 12573, 12813, 13053, 13309, 13565, 13821, 14093, 14365, 14637, 14925, 15213, 15502, 15806, 16110, 16414, 16734, 17054, 17390, 17726, 18062, 18414, 18766, 19134, 19502, 19886, 20270, 20670, 21070, 21486, 21902, 22334, 22766, 23214, 23662, 24126, 24590, 25070, 25551, 26047, 26559, 27071, 27599, 28143, 28687, 29247 }
|
||
};
|
||
|
||
return ac_qlookup[(bit_depth - 8) >> 1][clip_3<u8>(0, 255, b)];
|
||
}
|
||
|
||
u8 Decoder::get_base_quantizer_index(SegmentFeatureStatus alternative_quantizer_feature, bool should_use_absolute_segment_base_quantizer, u8 base_quantizer_index)
|
||
{
|
||
// The function get_qindex( ) returns the quantizer index for the current block and is specified by the following:
|
||
// − If seg_feature_active( SEG_LVL_ALT_Q ) is equal to 1 the following ordered steps apply:
|
||
if (alternative_quantizer_feature.enabled) {
|
||
// 1. Set the variable data equal to FeatureData[ segment_id ][ SEG_LVL_ALT_Q ].
|
||
auto data = alternative_quantizer_feature.value;
|
||
|
||
// 2. If segmentation_abs_or_delta_update is equal to 0, set data equal to base_q_idx + data
|
||
if (!should_use_absolute_segment_base_quantizer) {
|
||
data += base_quantizer_index;
|
||
}
|
||
|
||
// 3. Return Clip3( 0, 255, data ).
|
||
return clip_3<u8>(0, 255, data);
|
||
}
|
||
|
||
// − Otherwise, return base_q_idx.
|
||
return base_quantizer_index;
|
||
}
|
||
|
||
u16 Decoder::get_dc_quantizer(u8 bit_depth, u8 base, i8 delta)
|
||
{
|
||
// NOTE: Delta is selected by the caller based on whether it is for the Y or UV planes.
|
||
|
||
// The function get_dc_quant( plane ) returns the quantizer value for the dc coefficient for a particular plane and
|
||
// is derived as follows:
|
||
// − If plane is equal to 0, return dc_q( get_qindex( ) + delta_q_y_dc ).
|
||
// − Otherwise, return dc_q( get_qindex( ) + delta_q_uv_dc ).
|
||
return dc_q(bit_depth, static_cast<u8>(base + delta));
|
||
}
|
||
|
||
u16 Decoder::get_ac_quantizer(u8 bit_depth, u8 base, i8 delta)
|
||
{
|
||
// NOTE: Delta is selected by the caller based on whether it is for the Y or UV planes.
|
||
|
||
// The function get_ac_quant( plane ) returns the quantizer value for the ac coefficient for a particular plane and
|
||
// is derived as follows:
|
||
// − If plane is equal to 0, return ac_q( get_qindex( ) ).
|
||
// − Otherwise, return ac_q( get_qindex( ) + delta_q_uv_ac ).
|
||
return ac_q(bit_depth, static_cast<u8>(base + delta));
|
||
}
|
||
|
||
DecoderErrorOr<void> Decoder::reconstruct(u8 plane, BlockContext const& block_context, u32 transform_block_x, u32 transform_block_y, TransformSize transform_block_size, TransformSet transform_set)
|
||
{
|
||
// 8.6.2 Reconstruct process
|
||
|
||
// The variable n (specifying the base 2 logarithm of the width of the transform block) is set equal to 2 + txSz.
|
||
u8 log2_of_block_size = 2u + transform_block_size;
|
||
switch (log2_of_block_size) {
|
||
case 2:
|
||
return reconstruct_templated<2>(plane, block_context, transform_block_x, transform_block_y, transform_set);
|
||
break;
|
||
case 3:
|
||
return reconstruct_templated<3>(plane, block_context, transform_block_x, transform_block_y, transform_set);
|
||
break;
|
||
case 4:
|
||
return reconstruct_templated<4>(plane, block_context, transform_block_x, transform_block_y, transform_set);
|
||
break;
|
||
case 5:
|
||
return reconstruct_templated<5>(plane, block_context, transform_block_x, transform_block_y, transform_set);
|
||
break;
|
||
default:
|
||
VERIFY_NOT_REACHED();
|
||
}
|
||
}
|
||
|
||
template<u8 log2_of_block_size>
|
||
DecoderErrorOr<void> Decoder::reconstruct_templated(u8 plane, BlockContext const& block_context, u32 transform_block_x, u32 transform_block_y, TransformSet transform_set)
|
||
{
|
||
// 8.6.2 Reconstruct process, continued:
|
||
|
||
// The variable dqDenom is set equal to 2 if txSz is equal to Transform_32X32, otherwise dqDenom is set equal to 1.
|
||
constexpr Intermediate dq_denominator = log2_of_block_size == 5 ? 2 : 1;
|
||
// The variable n0 (specifying the width of the transform block) is set equal to 1 << n.
|
||
constexpr auto block_size = 1u << log2_of_block_size;
|
||
|
||
// 1. Dequant[ i ][ j ] is set equal to ( Tokens[ i * n0 + j ] * get_ac_quant( plane ) ) / dqDenom
|
||
// for i = 0..(n0-1), for j = 0..(n0-1)
|
||
Array<Intermediate, block_size * block_size> dequantized;
|
||
auto quantizers = block_context.frame_context.segment_quantizers[block_context.segment_id];
|
||
Intermediate ac_quant = plane == 0 ? quantizers.y_ac_quantizer : quantizers.uv_ac_quantizer;
|
||
auto const* tokens_raw = block_context.residual_tokens.data();
|
||
for (u32 i = 0; i < dequantized.size(); i++) {
|
||
dequantized[i] = (tokens_raw[i] * ac_quant) / dq_denominator;
|
||
}
|
||
|
||
// 2. Dequant[ 0 ][ 0 ] is set equal to ( Tokens[ 0 ] * get_dc_quant( plane ) ) / dqDenom
|
||
dequantized[0] = (block_context.residual_tokens[0] * (plane == 0 ? quantizers.y_dc_quantizer : quantizers.uv_dc_quantizer)) / dq_denominator;
|
||
|
||
// It is a requirement of bitstream conformance that the values written into the Dequant array in steps 1 and 2
|
||
// are representable by a signed integer with 8 + BitDepth bits.
|
||
// Note: Since bounds checks just ensure that we will not have resulting values that will overflow, it's non-fatal
|
||
// to allow these bounds to be violated. Therefore, we can avoid the performance cost here.
|
||
|
||
// 3. Invoke the 2D inverse transform block process defined in section 8.7.2 with the variable n as input.
|
||
// The inverse transform outputs are stored back to the Dequant buffer.
|
||
TRY(inverse_transform_2d<log2_of_block_size>(block_context, dequantized, transform_set));
|
||
|
||
// 4. CurrFrame[ plane ][ y + i ][ x + j ] is set equal to Clip1( CurrFrame[ plane ][ y + i ][ x + j ] + Dequant[ i ][ j ] )
|
||
// for i = 0..(n0-1) and j = 0..(n0-1).
|
||
auto& current_buffer = get_output_buffer(plane);
|
||
auto frame_size = block_context.frame_context.decoded_size(plane > 0);
|
||
auto width_in_frame_buffer = min(block_size, frame_size.width() - transform_block_x);
|
||
auto height_in_frame_buffer = min(block_size, frame_size.height() - transform_block_y);
|
||
|
||
for (auto i = 0u; i < height_in_frame_buffer; i++) {
|
||
for (auto j = 0u; j < width_in_frame_buffer; j++) {
|
||
auto index = (transform_block_y + i) * frame_size.width() + transform_block_x + j;
|
||
auto dequantized_value = dequantized[i * block_size + j];
|
||
current_buffer[index] = clip_1(block_context.frame_context.color_config.bit_depth, current_buffer[index] + dequantized_value);
|
||
}
|
||
}
|
||
|
||
return {};
|
||
}
|
||
|
||
inline DecoderErrorOr<void> Decoder::inverse_walsh_hadamard_transform(Span<Intermediate> data, u8 log2_of_block_size, u8 shift)
|
||
{
|
||
(void)data;
|
||
(void)shift;
|
||
// The input to this process is a variable shift that specifies the amount of pre-scaling.
|
||
// This process does an in-place transform of the array T (of length 4) by the following ordered steps:
|
||
if (1 << log2_of_block_size != 4)
|
||
return DecoderError::corrupted("Block size was not 4"sv);
|
||
|
||
return DecoderError::not_implemented();
|
||
}
|
||
|
||
inline i32 Decoder::cos64(u8 angle)
|
||
{
|
||
const i32 cos64_lookup[33] = { 16384, 16364, 16305, 16207, 16069, 15893, 15679, 15426, 15137, 14811, 14449, 14053, 13623, 13160, 12665, 12140, 11585, 11003, 10394, 9760, 9102, 8423, 7723, 7005, 6270, 5520, 4756, 3981, 3196, 2404, 1606, 804, 0 };
|
||
|
||
// 1. Set a variable angle2 equal to angle & 127.
|
||
angle &= 127;
|
||
// 2. If angle2 is greater than or equal to 0 and less than or equal to 32, return cos64_lookup[ angle2 ].
|
||
if (angle <= 32)
|
||
return cos64_lookup[angle];
|
||
// 3. If angle2 is greater than 32 and less than or equal to 64, return cos64_lookup[ 64 - angle2 ] * -1.
|
||
if (angle <= 64)
|
||
return -cos64_lookup[64 - angle];
|
||
// 4. If angle2 is greater than 64 and less than or equal to 96, return cos64_lookup[ angle2 - 64 ] * -1.
|
||
if (angle <= 96)
|
||
return -cos64_lookup[angle - 64];
|
||
// 5. Otherwise (if angle2 is greater than 96 and less than 128), return cos64_lookup[ 128 - angle2 ].
|
||
return cos64_lookup[128 - angle];
|
||
}
|
||
|
||
inline i32 Decoder::sin64(u8 angle)
|
||
{
|
||
if (angle < 32)
|
||
angle += 128;
|
||
return cos64(angle - 32u);
|
||
}
|
||
|
||
// (8.7.1.1) The function B( a, b, angle, 0 ) performs a butterfly rotation.
|
||
inline void Decoder::butterfly_rotation_in_place(Span<Intermediate> data, size_t index_a, size_t index_b, u8 angle, bool flip)
|
||
{
|
||
auto cos = cos64(angle);
|
||
auto sin = sin64(angle);
|
||
// 1. The variable x is set equal to T[ a ] * cos64( angle ) - T[ b ] * sin64( angle ).
|
||
i64 rotated_a = data[index_a] * cos - data[index_b] * sin;
|
||
// 2. The variable y is set equal to T[ a ] * sin64( angle ) + T[ b ] * cos64( angle ).
|
||
i64 rotated_b = data[index_a] * sin + data[index_b] * cos;
|
||
// 3. T[ a ] is set equal to Round2( x, 14 ).
|
||
data[index_a] = rounded_right_shift(rotated_a, 14);
|
||
// 4. T[ b ] is set equal to Round2( y, 14 ).
|
||
data[index_b] = rounded_right_shift(rotated_b, 14);
|
||
|
||
// The function B( a ,b, angle, 1 ) performs a butterfly rotation and flip specified by the following ordered steps:
|
||
// 1. The function B( a, b, angle, 0 ) is invoked.
|
||
// 2. The contents of T[ a ] and T[ b ] are exchanged.
|
||
if (flip)
|
||
swap(data[index_a], data[index_b]);
|
||
|
||
// It is a requirement of bitstream conformance that the values saved into the array T by this function are
|
||
// representable by a signed integer using 8 + BitDepth bits of precision.
|
||
// Note: Since bounds checks just ensure that we will not have resulting values that will overflow, it's non-fatal
|
||
// to allow these bounds to be violated. Therefore, we can avoid the performance cost here.
|
||
}
|
||
|
||
// (8.7.1.1) The function H( a, b, 0 ) performs a Hadamard rotation.
|
||
inline void Decoder::hadamard_rotation_in_place(Span<Intermediate> data, size_t index_a, size_t index_b, bool flip)
|
||
{
|
||
// The function H( a, b, 1 ) performs a Hadamard rotation with flipped indices and is specified as follows:
|
||
// 1. The function H( b, a, 0 ) is invoked.
|
||
if (flip)
|
||
swap(index_a, index_b);
|
||
|
||
// The function H( a, b, 0 ) performs a Hadamard rotation specified by the following ordered steps:
|
||
|
||
// 1. The variable x is set equal to T[ a ].
|
||
auto a_value = data[index_a];
|
||
// 2. The variable y is set equal to T[ b ].
|
||
auto b_value = data[index_b];
|
||
// 3. T[ a ] is set equal to x + y.
|
||
data[index_a] = a_value + b_value;
|
||
// 4. T[ b ] is set equal to x - y.
|
||
data[index_b] = a_value - b_value;
|
||
|
||
// It is a requirement of bitstream conformance that the values saved into the array T by this function are
|
||
// representable by a signed integer using 8 + BitDepth bits of precision.
|
||
// Note: Since bounds checks just ensure that we will not have resulting values that will overflow, it's non-fatal
|
||
// to allow these bounds to be violated. Therefore, we can avoid the performance cost here.
|
||
}
|
||
|
||
template<u8 log2_of_block_size>
|
||
inline DecoderErrorOr<void> Decoder::inverse_discrete_cosine_transform_array_permutation(Span<Intermediate> data)
|
||
{
|
||
static_assert(log2_of_block_size >= 2 && log2_of_block_size <= 5, "Block size out of range.");
|
||
|
||
constexpr u8 block_size = 1 << log2_of_block_size;
|
||
|
||
// This process performs an in-place permutation of the array T of length 2^n for 2 ≤ n ≤ 5 which is required before
|
||
// execution of the inverse DCT process.
|
||
if (log2_of_block_size < 2 || log2_of_block_size > 5)
|
||
return DecoderError::corrupted("Block size was out of range"sv);
|
||
|
||
// 1.1. A temporary array named copyT is set equal to T.
|
||
Array<Intermediate, block_size> data_copy;
|
||
AK::TypedTransfer<Intermediate>::copy(data_copy.data(), data.data(), block_size);
|
||
|
||
// 1.2. T[ i ] is set equal to copyT[ brev( n, i ) ] for i = 0..((1<<n) - 1).
|
||
for (auto i = 0u; i < block_size; i++)
|
||
data[i] = data_copy[brev<log2_of_block_size>(i)];
|
||
|
||
return {};
|
||
}
|
||
|
||
template<u8 log2_of_block_size>
|
||
ALWAYS_INLINE DecoderErrorOr<void> Decoder::inverse_discrete_cosine_transform(Span<Intermediate> data)
|
||
{
|
||
static_assert(log2_of_block_size >= 2 && log2_of_block_size <= 5, "Block size out of range.");
|
||
|
||
// 2.1. The variable n0 is set equal to 1<<n.
|
||
constexpr u8 block_size = 1 << log2_of_block_size;
|
||
|
||
// 8.7.1.3 Inverse DCT process
|
||
|
||
// 2.2. The variable n1 is set equal to 1<<(n-1).
|
||
constexpr u8 half_block_size = block_size >> 1;
|
||
// 2.3 The variable n2 is set equal to 1<<(n-2).
|
||
constexpr u8 quarter_block_size = half_block_size >> 1;
|
||
// 2.4 The variable n3 is set equal to 1<<(n-3).
|
||
constexpr u8 eighth_block_size = quarter_block_size >> 1;
|
||
|
||
// 2.5 If n is equal to 2, invoke B( 0, 1, 16, 1 ), otherwise recursively invoke the inverse DCT defined in this
|
||
// section with the variable n set equal to n - 1.
|
||
if constexpr (log2_of_block_size == 2)
|
||
butterfly_rotation_in_place(data, 0, 1, 16, true);
|
||
else
|
||
TRY(inverse_discrete_cosine_transform<log2_of_block_size - 1>(data));
|
||
|
||
// 2.6 Invoke B( n1+i, n0-1-i, 32-brev( 5, n1+i), 0 ) for i = 0..(n2-1).
|
||
for (auto i = 0u; i < quarter_block_size; i++) {
|
||
auto index = half_block_size + i;
|
||
butterfly_rotation_in_place(data, index, block_size - 1 - i, 32 - brev<5>(index), false);
|
||
}
|
||
|
||
// 2.7 If n is greater than or equal to 3:
|
||
if constexpr (log2_of_block_size >= 3) {
|
||
// a. Invoke H( n1+4*i+2*j, n1+1+4*i+2*j, j ) for i = 0..(n3-1), j = 0..1.
|
||
for (auto i = 0u; i < eighth_block_size; i++) {
|
||
for (auto j = 0u; j < 2; j++) {
|
||
auto index = half_block_size + (4 * i) + (2 * j);
|
||
hadamard_rotation_in_place(data, index, index + 1, j);
|
||
}
|
||
}
|
||
}
|
||
|
||
// 4. If n is equal to 5:
|
||
if constexpr (log2_of_block_size == 5) {
|
||
// a. Invoke B( n0-n+3-n2*j-4*i, n1+n-4+n2*j+4*i, 28-16*i+56*j, 1 ) for i = 0..1, j = 0..1.
|
||
for (auto i = 0u; i < 2; i++) {
|
||
for (auto j = 0u; j < 2; j++) {
|
||
auto index_a = block_size - log2_of_block_size + 3 - (quarter_block_size * j) - (4 * i);
|
||
auto index_b = half_block_size + log2_of_block_size - 4 + (quarter_block_size * j) + (4 * i);
|
||
auto angle = 28 - (16 * i) + (56 * j);
|
||
butterfly_rotation_in_place(data, index_a, index_b, angle, true);
|
||
}
|
||
}
|
||
|
||
// b. Invoke H( n1+n3*j+i, n1+n2-5+n3*j-i, j&1 ) for i = 0..1, j = 0..3.
|
||
for (auto i = 0u; i < 2; i++) {
|
||
for (auto j = 0u; j < 4; j++) {
|
||
auto index_a = half_block_size + (eighth_block_size * j) + i;
|
||
auto index_b = half_block_size + quarter_block_size - 5 + (eighth_block_size * j) - i;
|
||
hadamard_rotation_in_place(data, index_a, index_b, (j & 1) != 0);
|
||
}
|
||
}
|
||
}
|
||
|
||
// 5. If n is greater than or equal to 4:
|
||
if constexpr (log2_of_block_size >= 4) {
|
||
// a. Invoke B( n0-n+2-i-n2*j, n1+n-3+i+n2*j, 24+48*j, 1 ) for i = 0..(n==5), j = 0..1.
|
||
for (auto i = 0u; i <= (log2_of_block_size == 5); i++) {
|
||
for (auto j = 0u; j < 2; j++) {
|
||
auto index_a = block_size - log2_of_block_size + 2 - i - (quarter_block_size * j);
|
||
auto index_b = half_block_size + log2_of_block_size - 3 + i + (quarter_block_size * j);
|
||
butterfly_rotation_in_place(data, index_a, index_b, 24 + (48 * j), true);
|
||
}
|
||
}
|
||
|
||
// b. Invoke H( n1+n2*j+i, n1+n2-1+n2*j-i, j&1 ) for i = 0..(2n-7), j = 0..1.
|
||
for (auto i = 0u; i < (2 * log2_of_block_size) - 6u; i++) {
|
||
for (auto j = 0u; j < 2; j++) {
|
||
auto index_a = half_block_size + (quarter_block_size * j) + i;
|
||
auto index_b = half_block_size + quarter_block_size - 1 + (quarter_block_size * j) - i;
|
||
hadamard_rotation_in_place(data, index_a, index_b, (j & 1) != 0);
|
||
}
|
||
}
|
||
}
|
||
|
||
// 6. If n is greater than or equal to 3:
|
||
if constexpr (log2_of_block_size >= 3) {
|
||
// a. Invoke B( n0-n3-1-i, n1+n3+i, 16, 1 ) for i = 0..(n3-1).
|
||
for (auto i = 0u; i < eighth_block_size; i++) {
|
||
auto index_a = block_size - eighth_block_size - 1 - i;
|
||
auto index_b = half_block_size + eighth_block_size + i;
|
||
butterfly_rotation_in_place(data, index_a, index_b, 16, true);
|
||
}
|
||
}
|
||
|
||
// 7. Invoke H( i, n0-1-i, 0 ) for i = 0..(n1-1).
|
||
for (auto i = 0u; i < half_block_size; i++)
|
||
hadamard_rotation_in_place(data, i, block_size - 1 - i, false);
|
||
|
||
return {};
|
||
}
|
||
|
||
template<u8 log2_of_block_size>
|
||
inline void Decoder::inverse_asymmetric_discrete_sine_transform_input_array_permutation(Span<Intermediate> data)
|
||
{
|
||
// The variable n0 is set equal to 1<<n.
|
||
constexpr auto block_size = 1u << log2_of_block_size;
|
||
// The variable n1 is set equal to 1<<(n-1).
|
||
// We can iterate by 2 at a time instead of taking half block size.
|
||
|
||
// A temporary array named copyT is set equal to T.
|
||
Array<Intermediate, block_size> data_copy;
|
||
AK::TypedTransfer<Intermediate>::copy(data_copy.data(), data.data(), block_size);
|
||
|
||
// The values at even locations T[ 2 * i ] are set equal to copyT[ n0 - 1 - 2 * i ] for i = 0..(n1-1).
|
||
// The values at odd locations T[ 2 * i + 1 ] are set equal to copyT[ 2 * i ] for i = 0..(n1-1).
|
||
for (auto i = 0u; i < block_size; i += 2) {
|
||
data[i] = data_copy[block_size - 1 - i];
|
||
data[i + 1] = data_copy[i];
|
||
}
|
||
}
|
||
|
||
template<u8 log2_of_block_size>
|
||
inline void Decoder::inverse_asymmetric_discrete_sine_transform_output_array_permutation(Span<Intermediate> data)
|
||
{
|
||
auto block_size = 1u << log2_of_block_size;
|
||
|
||
// A temporary array named copyT is set equal to T.
|
||
Array<Intermediate, maximum_transform_size> data_copy;
|
||
AK::TypedTransfer<Intermediate>::copy(data_copy.data(), data.data(), block_size);
|
||
|
||
// The permutation depends on n as follows:
|
||
if (log2_of_block_size == 4) {
|
||
// − If n is equal to 4,
|
||
// T[ 8*a + 4*b + 2*c + d ] is set equal to copyT[ 8*(d^c) + 4*(c^b) + 2*(b^a) + a ] for a = 0..1
|
||
// and b = 0..1 and c = 0..1 and d = 0..1.
|
||
for (auto a = 0u; a < 2; a++)
|
||
for (auto b = 0u; b < 2; b++)
|
||
for (auto c = 0u; c < 2; c++)
|
||
for (auto d = 0u; d < 2; d++)
|
||
data[(8 * a) + (4 * b) + (2 * c) + d] = data_copy[8 * (d ^ c) + 4 * (c ^ b) + 2 * (b ^ a) + a];
|
||
} else {
|
||
VERIFY(log2_of_block_size == 3);
|
||
// − Otherwise (n is equal to 3),
|
||
// T[ 4*a + 2*b + c ] is set equal to copyT[ 4*(c^b) + 2*(b^a) + a ] for a = 0..1 and
|
||
// b = 0..1 and c = 0..1.
|
||
for (auto a = 0u; a < 2; a++)
|
||
for (auto b = 0u; b < 2; b++)
|
||
for (auto c = 0u; c < 2; c++)
|
||
data[4 * a + 2 * b + c] = data_copy[4 * (c ^ b) + 2 * (b ^ a) + a];
|
||
}
|
||
}
|
||
|
||
inline void Decoder::inverse_asymmetric_discrete_sine_transform_4(Span<Intermediate> data)
|
||
{
|
||
VERIFY(data.size() == 4);
|
||
const i64 sinpi_1_9 = 5283;
|
||
const i64 sinpi_2_9 = 9929;
|
||
const i64 sinpi_3_9 = 13377;
|
||
const i64 sinpi_4_9 = 15212;
|
||
|
||
// Steps are derived from pseudocode in (8.7.1.6):
|
||
// s0 = SINPI_1_9 * T[ 0 ]
|
||
i64 s0 = sinpi_1_9 * data[0];
|
||
// s1 = SINPI_2_9 * T[ 0 ]
|
||
i64 s1 = sinpi_2_9 * data[0];
|
||
// s2 = SINPI_3_9 * T[ 1 ]
|
||
i64 s2 = sinpi_3_9 * data[1];
|
||
// s3 = SINPI_4_9 * T[ 2 ]
|
||
i64 s3 = sinpi_4_9 * data[2];
|
||
// s4 = SINPI_1_9 * T[ 2 ]
|
||
i64 s4 = sinpi_1_9 * data[2];
|
||
// s5 = SINPI_2_9 * T[ 3 ]
|
||
i64 s5 = sinpi_2_9 * data[3];
|
||
// s6 = SINPI_4_9 * T[ 3 ]
|
||
i64 s6 = sinpi_4_9 * data[3];
|
||
// v = T[ 0 ] - T[ 2 ] + T[ 3 ]
|
||
// s7 = SINPI_3_9 * v
|
||
i64 s7 = sinpi_3_9 * (data[0] - data[2] + data[3]);
|
||
|
||
// x0 = s0 + s3 + s5
|
||
auto x0 = s0 + s3 + s5;
|
||
// x1 = s1 - s4 - s6
|
||
auto x1 = s1 - s4 - s6;
|
||
// x2 = s7
|
||
auto x2 = s7;
|
||
// x3 = s2
|
||
auto x3 = s2;
|
||
|
||
// s0 = x0 + x3
|
||
s0 = x0 + x3;
|
||
// s1 = x1 + x3
|
||
s1 = x1 + x3;
|
||
// s2 = x2
|
||
s2 = x2;
|
||
// s3 = x0 + x1 - x3
|
||
s3 = x0 + x1 - x3;
|
||
|
||
// T[ 0 ] = Round2( s0, 14 )
|
||
data[0] = rounded_right_shift(s0, 14);
|
||
// T[ 1 ] = Round2( s1, 14 )
|
||
data[1] = rounded_right_shift(s1, 14);
|
||
// T[ 2 ] = Round2( s2, 14 )
|
||
data[2] = rounded_right_shift(s2, 14);
|
||
// T[ 3 ] = Round2( s3, 14 )
|
||
data[3] = rounded_right_shift(s3, 14);
|
||
|
||
// (8.7.1.1) The inverse asymmetric discrete sine transforms also make use of an intermediate array named S.
|
||
// The values in this array require higher precision to avoid overflow. Using signed integers with 24 +
|
||
// BitDepth bits of precision is enough to avoid overflow.
|
||
// Note: Since bounds checks just ensure that we will not have resulting values that will overflow, it's non-fatal
|
||
// to allow these bounds to be violated. Therefore, we can avoid the performance cost here.
|
||
}
|
||
|
||
// The function SB( a, b, angle, 0 ) performs a butterfly rotation.
|
||
// Spec defines the source as array T, and the destination array as S.
|
||
template<typename S, typename D>
|
||
inline void Decoder::butterfly_rotation(Span<S> source, Span<D> destination, size_t index_a, size_t index_b, u8 angle, bool flip)
|
||
{
|
||
// The function SB( a, b, angle, 0 ) performs a butterfly rotation according to the following ordered steps:
|
||
auto cos = cos64(angle);
|
||
auto sin = sin64(angle);
|
||
// Expand to the destination buffer's precision.
|
||
D a = source[index_a];
|
||
D b = source[index_b];
|
||
// 1. S[ a ] is set equal to T[ a ] * cos64( angle ) - T[ b ] * sin64( angle ).
|
||
destination[index_a] = a * cos - b * sin;
|
||
// 2. S[ b ] is set equal to T[ a ] * sin64( angle ) + T[ b ] * cos64( angle ).
|
||
destination[index_b] = a * sin + b * cos;
|
||
|
||
// The function SB( a, b, angle, 1 ) performs a butterfly rotation and flip according to the following ordered steps:
|
||
// 1. The function SB( a, b, angle, 0 ) is invoked.
|
||
// 2. The contents of S[ a ] and S[ b ] are exchanged.
|
||
if (flip)
|
||
swap(destination[index_a], destination[index_b]);
|
||
}
|
||
|
||
// The function SH( a, b ) performs a Hadamard rotation and rounding.
|
||
// Spec defines the source array as S, and the destination array as T.
|
||
template<typename S, typename D>
|
||
inline void Decoder::hadamard_rotation(Span<S> source, Span<D> destination, size_t index_a, size_t index_b)
|
||
{
|
||
// Keep the source buffer's precision until rounding.
|
||
S a = source[index_a];
|
||
S b = source[index_b];
|
||
// 1. T[ a ] is set equal to Round2( S[ a ] + S[ b ], 14 ).
|
||
destination[index_a] = rounded_right_shift(a + b, 14);
|
||
// 2. T[ b ] is set equal to Round2( S[ a ] - S[ b ], 14 ).
|
||
destination[index_b] = rounded_right_shift(a - b, 14);
|
||
}
|
||
|
||
inline DecoderErrorOr<void> Decoder::inverse_asymmetric_discrete_sine_transform_8(Span<Intermediate> data)
|
||
{
|
||
VERIFY(data.size() == 8);
|
||
// This process does an in-place transform of the array T using:
|
||
|
||
// A higher precision array S for intermediate results.
|
||
// (8.7.1.1) NOTE - The values in array S require higher precision to avoid overflow. Using signed integers with
|
||
// 24 + BitDepth bits of precision is enough to avoid overflow.
|
||
Array<i64, 8> high_precision_temp;
|
||
|
||
// The following ordered steps apply:
|
||
|
||
// 1. Invoke the ADST input array permutation process specified in section 8.7.1.4 with the input variable n set
|
||
// equal to 3.
|
||
inverse_asymmetric_discrete_sine_transform_input_array_permutation<3>(data);
|
||
|
||
// 2. Invoke SB( 2*i, 1+2*i, 30-8*i, 1 ) for i = 0..3.
|
||
for (auto i = 0u; i < 4; i++)
|
||
butterfly_rotation(data, high_precision_temp.span(), 2 * i, 1 + (2 * i), 30 - (8 * i), true);
|
||
|
||
// 3. Invoke SH( i, 4+i ) for i = 0..3.
|
||
for (auto i = 0u; i < 4; i++)
|
||
hadamard_rotation(high_precision_temp.span(), data, i, 4 + i);
|
||
|
||
// 4. Invoke SB( 4+3*i, 5+i, 24-16*i, 1 ) for i = 0..1.
|
||
for (auto i = 0u; i < 2; i++)
|
||
butterfly_rotation(data, high_precision_temp.span(), 4 + (3 * i), 5 + i, 24 - (16 * i), true);
|
||
// 5. Invoke SH( 4+i, 6+i ) for i = 0..1.
|
||
for (auto i = 0u; i < 2; i++)
|
||
hadamard_rotation(high_precision_temp.span(), data, 4 + i, 6 + i);
|
||
|
||
// 6. Invoke H( i, 2+i, 0 ) for i = 0..1.
|
||
for (auto i = 0u; i < 2; i++)
|
||
hadamard_rotation_in_place(data, i, 2 + i, false);
|
||
|
||
// 7. Invoke B( 2+4*i, 3+4*i, 16, 1 ) for i = 0..1.
|
||
for (auto i = 0u; i < 2; i++)
|
||
butterfly_rotation_in_place(data, 2 + (4 * i), 3 + (4 * i), 16, true);
|
||
|
||
// 8. Invoke the ADST output array permutation process specified in section 8.7.1.5 with the input variable n
|
||
// set equal to 3.
|
||
inverse_asymmetric_discrete_sine_transform_output_array_permutation<3>(data);
|
||
|
||
// 9. Set T[ 1+2*i ] equal to -T[ 1+2*i ] for i = 0..3.
|
||
for (auto i = 0u; i < 4; i++) {
|
||
auto index = 1 + (2 * i);
|
||
data[index] = -data[index];
|
||
}
|
||
return {};
|
||
}
|
||
|
||
inline DecoderErrorOr<void> Decoder::inverse_asymmetric_discrete_sine_transform_16(Span<Intermediate> data)
|
||
{
|
||
VERIFY(data.size() == 16);
|
||
// This process does an in-place transform of the array T using:
|
||
|
||
// A higher precision array S for intermediate results.
|
||
// (8.7.1.1) The inverse asymmetric discrete sine transforms also make use of an intermediate array named S.
|
||
// The values in this array require higher precision to avoid overflow. Using signed integers with 24 +
|
||
// BitDepth bits of precision is enough to avoid overflow.
|
||
Array<i64, 16> high_precision_temp;
|
||
|
||
// The following ordered steps apply:
|
||
|
||
// 1. Invoke the ADST input array permutation process specified in section 8.7.1.4 with the input variable n set
|
||
// equal to 4.
|
||
inverse_asymmetric_discrete_sine_transform_input_array_permutation<4>(data);
|
||
|
||
// 2. Invoke SB( 2*i, 1+2*i, 31-4*i, 1 ) for i = 0..7.
|
||
for (auto i = 0u; i < 8; i++)
|
||
butterfly_rotation(data, high_precision_temp.span(), 2 * i, 1 + (2 * i), 31 - (4 * i), true);
|
||
// 3. Invoke SH( i, 8+i ) for i = 0..7.
|
||
for (auto i = 0u; i < 8; i++)
|
||
hadamard_rotation(high_precision_temp.span(), data, i, 8 + i);
|
||
|
||
// 4. Invoke SB( 8+2*i, 9+2*i, 28-16*i, 1 ) for i = 0..3.
|
||
for (auto i = 0u; i < 4; i++)
|
||
butterfly_rotation(data, high_precision_temp.span(), 8 + (2 * i), 9 + (2 * i), 128 + 28 - (16 * i), true);
|
||
// 5. Invoke SH( 8+i, 12+i ) for i = 0..3.
|
||
for (auto i = 0u; i < 4; i++)
|
||
hadamard_rotation(high_precision_temp.span(), data, 8 + i, 12 + i);
|
||
|
||
// 6. Invoke H( i, 4+i, 0 ) for i = 0..3.
|
||
for (auto i = 0u; i < 4; i++)
|
||
hadamard_rotation_in_place(data, i, 4 + i, false);
|
||
|
||
// 7. Invoke SB( 4+8*i+3*j, 5+8*i+j, 24-16*j, 1 ) for i = 0..1, for j = 0..1.
|
||
for (auto i = 0u; i < 2; i++)
|
||
for (auto j = 0u; j < 2; j++)
|
||
butterfly_rotation(data, high_precision_temp.span(), 4 + (8 * i) + (3 * j), 5 + (8 * i) + j, 24 - (16 * j), true);
|
||
// 8. Invoke SH( 4+8*j+i, 6+8*j+i ) for i = 0..1, j = 0..1.
|
||
for (auto i = 0u; i < 2; i++)
|
||
for (auto j = 0u; j < 2; j++)
|
||
hadamard_rotation(high_precision_temp.span(), data, 4 + (8 * j) + i, 6 + (8 * j) + i);
|
||
|
||
// 9. Invoke H( 8*j+i, 2+8*j+i, 0 ) for i = 0..1, for j = 0..1.
|
||
for (auto i = 0u; i < 2; i++)
|
||
for (auto j = 0u; j < 2; j++)
|
||
hadamard_rotation_in_place(data, (8 * j) + i, 2 + (8 * j) + i, false);
|
||
// 10. Invoke B( 2+4*j+8*i, 3+4*j+8*i, 48+64*(i^j), 0 ) for i = 0..1, for j = 0..1.
|
||
for (auto i = 0u; i < 2; i++)
|
||
for (auto j = 0u; j < 2; j++)
|
||
butterfly_rotation_in_place(data, 2 + (4 * j) + (8 * i), 3 + (4 * j) + (8 * i), 48 + (64 * (i ^ j)), false);
|
||
|
||
// 11. Invoke the ADST output array permutation process specified in section 8.7.1.5 with the input variable n
|
||
// set equal to 4.
|
||
inverse_asymmetric_discrete_sine_transform_output_array_permutation<4>(data);
|
||
|
||
// 12. Set T[ 1+12*j+2*i ] equal to -T[ 1+12*j+2*i ] for i = 0..1, for j = 0..1.
|
||
for (auto i = 0u; i < 2; i++) {
|
||
for (auto j = 0u; j < 2; j++) {
|
||
auto index = 1 + (12 * j) + (2 * i);
|
||
data[index] = -data[index];
|
||
}
|
||
}
|
||
return {};
|
||
}
|
||
|
||
template<u8 log2_of_block_size>
|
||
inline DecoderErrorOr<void> Decoder::inverse_asymmetric_discrete_sine_transform(Span<Intermediate> data)
|
||
{
|
||
// 8.7.1.9 Inverse ADST Process
|
||
|
||
// This process performs an in-place inverse ADST process on the array T of size 2^n for 2 ≤ n ≤ 4.
|
||
if constexpr (log2_of_block_size < 2 || log2_of_block_size > 4)
|
||
return DecoderError::corrupted("Block size was out of range"sv);
|
||
|
||
// The process to invoke depends on n as follows:
|
||
if constexpr (log2_of_block_size == 2) {
|
||
// − If n is equal to 2, invoke the Inverse ADST4 process specified in section 8.7.1.6.
|
||
inverse_asymmetric_discrete_sine_transform_4(data);
|
||
return {};
|
||
}
|
||
if constexpr (log2_of_block_size == 3) {
|
||
// − Otherwise if n is equal to 3, invoke the Inverse ADST8 process specified in section 8.7.1.7.
|
||
return inverse_asymmetric_discrete_sine_transform_8(data);
|
||
}
|
||
// − Otherwise (n is equal to 4), invoke the Inverse ADST16 process specified in section 8.7.1.8.
|
||
return inverse_asymmetric_discrete_sine_transform_16(data);
|
||
}
|
||
|
||
template<u8 log2_of_block_size>
|
||
ALWAYS_INLINE DecoderErrorOr<void> Decoder::inverse_transform_2d(BlockContext const& block_context, Span<Intermediate> dequantized, TransformSet transform_set)
|
||
{
|
||
static_assert(log2_of_block_size >= 2 && log2_of_block_size <= 5);
|
||
|
||
// This process performs a 2D inverse transform for an array of size 2^n by 2^n stored in the 2D array Dequant.
|
||
// The input to this process is a variable n (log2_of_block_size) that specifies the base 2 logarithm of the width of the transform.
|
||
|
||
// 1. Set the variable n0 (block_size) equal to 1 << n.
|
||
constexpr auto block_size = 1u << log2_of_block_size;
|
||
|
||
Array<Intermediate, block_size * block_size> row_array;
|
||
Span<Intermediate> row = row_array.span().trim(block_size);
|
||
|
||
// 2. The row transforms with i = 0..(n0-1) are applied as follows:
|
||
for (auto i = 0u; i < block_size; i++) {
|
||
// 1. Set T[ j ] equal to Dequant[ i ][ j ] for j = 0..(n0-1).
|
||
for (auto j = 0u; j < block_size; j++)
|
||
row[j] = dequantized[i * block_size + j];
|
||
|
||
// 2. If Lossless is equal to 1, invoke the Inverse WHT process as specified in section 8.7.1.10 with shift equal
|
||
// to 2.
|
||
if (block_context.frame_context.lossless) {
|
||
TRY(inverse_walsh_hadamard_transform(row, log2_of_block_size, 2));
|
||
continue;
|
||
}
|
||
switch (transform_set.second_transform) {
|
||
case TransformType::DCT:
|
||
// Otherwise, if TxType is equal to DCT_DCT or TxType is equal to ADST_DCT, apply an inverse DCT as
|
||
// follows:
|
||
// 1. Invoke the inverse DCT permutation process as specified in section 8.7.1.2 with the input variable n.
|
||
TRY(inverse_discrete_cosine_transform_array_permutation<log2_of_block_size>(row));
|
||
// 2. Invoke the inverse DCT process as specified in section 8.7.1.3 with the input variable n.
|
||
TRY(inverse_discrete_cosine_transform<log2_of_block_size>(row));
|
||
break;
|
||
case TransformType::ADST:
|
||
// 4. Otherwise (TxType is equal to DCT_ADST or TxType is equal to ADST_ADST), invoke the inverse ADST
|
||
// process as specified in section 8.7.1.9 with input variable n.
|
||
TRY(inverse_asymmetric_discrete_sine_transform<log2_of_block_size>(row));
|
||
break;
|
||
default:
|
||
return DecoderError::corrupted("Unknown tx_type"sv);
|
||
}
|
||
|
||
// 5. Set Dequant[ i ][ j ] equal to T[ j ] for j = 0..(n0-1).
|
||
for (auto j = 0u; j < block_size; j++)
|
||
dequantized[i * block_size + j] = row[j];
|
||
}
|
||
|
||
Array<Intermediate, block_size * block_size> column_array;
|
||
auto column = column_array.span().trim(block_size);
|
||
|
||
// 3. The column transforms with j = 0..(n0-1) are applied as follows:
|
||
for (auto j = 0u; j < block_size; j++) {
|
||
// 1. Set T[ i ] equal to Dequant[ i ][ j ] for i = 0..(n0-1).
|
||
for (auto i = 0u; i < block_size; i++)
|
||
column[i] = dequantized[i * block_size + j];
|
||
|
||
// 2. If Lossless is equal to 1, invoke the Inverse WHT process as specified in section 8.7.1.10 with shift equal
|
||
// to 0.
|
||
if (block_context.frame_context.lossless) {
|
||
TRY(inverse_walsh_hadamard_transform(column, log2_of_block_size, 2));
|
||
continue;
|
||
}
|
||
switch (transform_set.first_transform) {
|
||
case TransformType::DCT:
|
||
// Otherwise, if TxType is equal to DCT_DCT or TxType is equal to DCT_ADST, apply an inverse DCT as
|
||
// follows:
|
||
// 1. Invoke the inverse DCT permutation process as specified in section 8.7.1.2 with the input variable n.
|
||
TRY(inverse_discrete_cosine_transform_array_permutation<log2_of_block_size>(column));
|
||
// 2. Invoke the inverse DCT process as specified in section 8.7.1.3 with the input variable n.
|
||
TRY(inverse_discrete_cosine_transform<log2_of_block_size>(column));
|
||
break;
|
||
case TransformType::ADST:
|
||
// 4. Otherwise (TxType is equal to ADST_DCT or TxType is equal to ADST_ADST), invoke the inverse ADST
|
||
// process as specified in section 8.7.1.9 with input variable n.
|
||
TRY(inverse_asymmetric_discrete_sine_transform<log2_of_block_size>(column));
|
||
break;
|
||
default:
|
||
VERIFY_NOT_REACHED();
|
||
}
|
||
|
||
// 5. If Lossless is equal to 1, set Dequant[ i ][ j ] equal to T[ i ] for i = 0..(n0-1).
|
||
for (auto i = 0u; i < block_size; i++)
|
||
dequantized[i * block_size + j] = column[i];
|
||
|
||
// 6. Otherwise (Lossless is equal to 0), set Dequant[ i ][ j ] equal to Round2( T[ i ], Min( 6, n + 2 ) )
|
||
// for i = 0..(n0-1).
|
||
if (!block_context.frame_context.lossless) {
|
||
for (auto i = 0u; i < block_size; i++) {
|
||
auto index = i * block_size + j;
|
||
dequantized[index] = rounded_right_shift(dequantized[index], min(6, log2_of_block_size + 2));
|
||
}
|
||
}
|
||
}
|
||
|
||
return {};
|
||
}
|
||
|
||
DecoderErrorOr<void> Decoder::update_reference_frames(FrameContext const& frame_context)
|
||
{
|
||
// This process is invoked as the final step in decoding a frame.
|
||
// The inputs to this process are the samples in the current frame CurrFrame[ plane ][ x ][ y ].
|
||
// The output from this process is an updated set of reference frames and previous motion vectors.
|
||
// The following ordered steps apply:
|
||
|
||
// 1. For each value of i from 0 to NUM_REF_FRAMES - 1, the following applies if bit i of refresh_frame_flags
|
||
// is equal to 1 (i.e. if (refresh_frame_flags>>i)&1 is equal to 1):
|
||
for (u8 i = 0; i < NUM_REF_FRAMES; i++) {
|
||
if (frame_context.should_update_reference_frame_at_index(i)) {
|
||
auto& reference_frame = m_parser->m_reference_frames[i];
|
||
|
||
// − RefFrameWidth[ i ] is set equal to FrameWidth.
|
||
// − RefFrameHeight[ i ] is set equal to FrameHeight.
|
||
reference_frame.size = frame_context.size();
|
||
// − RefSubsamplingX[ i ] is set equal to subsampling_x.
|
||
reference_frame.subsampling_x = frame_context.color_config.subsampling_x;
|
||
// − RefSubsamplingY[ i ] is set equal to subsampling_y.
|
||
reference_frame.subsampling_y = frame_context.color_config.subsampling_y;
|
||
// − RefBitDepth[ i ] is set equal to BitDepth.
|
||
reference_frame.bit_depth = frame_context.color_config.bit_depth;
|
||
|
||
// − FrameStore[ i ][ 0 ][ y ][ x ] is set equal to CurrFrame[ 0 ][ y ][ x ] for x = 0..FrameWidth-1, for y =
|
||
// 0..FrameHeight-1.
|
||
// − FrameStore[ i ][ plane ][ y ][ x ] is set equal to CurrFrame[ plane ][ y ][ x ] for plane = 1..2, for x =
|
||
// 0..((FrameWidth+subsampling_x) >> subsampling_x)-1, for y = 0..((FrameHeight+subsampling_y) >>
|
||
// subsampling_y)-1.
|
||
|
||
// FIXME: Frame width is not equal to the buffer's stride. If we store the stride of the buffer with the reference
|
||
// frame, we can just copy the framebuffer data instead. Alternatively, we should crop the output framebuffer.
|
||
for (auto plane = 0u; plane < 3; plane++) {
|
||
auto width = frame_context.size().width();
|
||
auto height = frame_context.size().height();
|
||
auto stride = frame_context.decoded_size(plane > 0).width();
|
||
if (plane > 0) {
|
||
width = y_size_to_uv_size(frame_context.color_config.subsampling_x, width);
|
||
height = y_size_to_uv_size(frame_context.color_config.subsampling_y, height);
|
||
}
|
||
|
||
auto const& original_buffer = get_output_buffer(plane);
|
||
auto& frame_store_buffer = reference_frame.frame_planes[plane];
|
||
auto frame_store_width = width + MV_BORDER * 2;
|
||
auto frame_store_height = height + MV_BORDER * 2;
|
||
frame_store_buffer.resize_and_keep_capacity(frame_store_width * frame_store_height);
|
||
|
||
VERIFY(original_buffer.size() >= width * height);
|
||
for (auto destination_y = 0u; destination_y < frame_store_height; destination_y++) {
|
||
// Offset the source row by the motion vector border and then clamp it to the range of 0...height.
|
||
// This will create an extended border on the top and bottom of the reference frame to avoid having to bounds check
|
||
// inter-prediction.
|
||
auto source_y = min(destination_y >= MV_BORDER ? destination_y - MV_BORDER : 0, height - 1);
|
||
auto const* source = &original_buffer[source_y * stride];
|
||
auto* destination = &frame_store_buffer[destination_y * frame_store_width + MV_BORDER];
|
||
AK::TypedTransfer<RemoveReference<decltype(*destination)>>::copy(destination, source, width);
|
||
}
|
||
|
||
for (auto destination_y = 0u; destination_y < frame_store_height; destination_y++) {
|
||
// Stretch the leftmost samples out into the border.
|
||
auto sample = frame_store_buffer[destination_y * frame_store_width + MV_BORDER];
|
||
|
||
for (auto destination_x = 0u; destination_x < MV_BORDER; destination_x++) {
|
||
frame_store_buffer[destination_y * frame_store_width + destination_x] = sample;
|
||
}
|
||
|
||
// Stretch the rightmost samples out into the border.
|
||
sample = frame_store_buffer[destination_y * frame_store_width + MV_BORDER + width - 1];
|
||
|
||
for (auto destination_x = MV_BORDER + width; destination_x < frame_store_width; destination_x++) {
|
||
frame_store_buffer[destination_y * frame_store_width + destination_x] = sample;
|
||
}
|
||
}
|
||
}
|
||
}
|
||
}
|
||
|
||
// 2. If show_existing_frame is equal to 0, the following applies:
|
||
if (!frame_context.shows_existing_frame()) {
|
||
DECODER_TRY_ALLOC(m_parser->m_previous_block_contexts.try_resize_to_match_other_vector2d(frame_context.block_contexts()));
|
||
// − PrevRefFrames[ row ][ col ][ list ] is set equal to RefFrames[ row ][ col ][ list ] for row = 0..MiRows-1,
|
||
// for col = 0..MiCols-1, for list = 0..1.
|
||
// − PrevMvs[ row ][ col ][ list ][ comp ] is set equal to Mvs[ row ][ col ][ list ][ comp ] for row = 0..MiRows-1,
|
||
// for col = 0..MiCols-1, for list = 0..1, for comp = 0..1.
|
||
// And from decode_frame():
|
||
// - If all of the following conditions are true, PrevSegmentIds[ row ][ col ] is set equal to
|
||
// SegmentIds[ row ][ col ] for row = 0..MiRows-1, for col = 0..MiCols-1:
|
||
// − show_existing_frame is equal to 0,
|
||
// − segmentation_enabled is equal to 1,
|
||
// − segmentation_update_map is equal to 1.
|
||
bool keep_segment_ids = !frame_context.shows_existing_frame() && frame_context.segmentation_enabled && frame_context.use_full_segment_id_tree;
|
||
frame_context.block_contexts().copy_to(m_parser->m_previous_block_contexts, [keep_segment_ids](FrameBlockContext context) {
|
||
auto persistent_context = PersistentBlockContext(context);
|
||
if (!keep_segment_ids)
|
||
persistent_context.segment_id = 0;
|
||
return persistent_context;
|
||
});
|
||
}
|
||
|
||
return {};
|
||
}
|
||
|
||
}
|